1. Introduction
Wearable biosensors in health assessments have stimulated technological development in many fields ranging from materials science to biomedicine[1-4]. Such health assessments, conducted through the dynamic and non-invasive measurements of biomarkers in sweat, tear, human saliva, or other biological fluids, provide insights into continuous and real-time physiological information. Research on wearable biosensors could be dated back to 2009 when Morris first reported a sweat analysis device that could collect sweat and perform pH analysis[5], which successfully demonstrated the feasibility of applying wearable sensors for the analysis of human metabolites. To date, recent research advances in materials synthesis and structural design have made significant progress in wearable biosensors, providing alternatives to conventional bulky devices[6-8] for clinical applications. Especially for non-invasive body computing[9-11], namely in the area of sports management[12, 13], health monitoring[14, 15], drug monitoring[16], and elderly care[17], wearable biosensors have delivered the attractive form factors including flexibility, lightweight and facile integration with data analysis/transmission modules.
To satisfy the requirement of wearing comfort, convenience of use, and conformableness to the human body, current wearable devices for human health detection are usually developed as a portable or skin-attachable format, and then attached to the wrist, arm, eyes, and even inside of the mouth of the human body for the detection of human sweat, tears, and saliva. Wrist watches[18], skin-attachable patches[19, 20], gloves[21], optical lenses[22], and mouth guards[11] have been extensively studied. Since sweat contains a large variety of biomarkers and its samples are easy to collect, sweat is widely regarded as the most meaningful and reliable analyte among various types of biological fluids. For example, the concentration of Na+ in sweat is highly related to the level in blood[23] so that sweat sensors with selective sensing ability of Na+ can exhibit promising applications for cystic fibrosis and cardiovascular risk diagnosis[16, 24, 25]. By analyzing cortisol, vitamin C, and glucose in the human sweat, one can assess physiological stress dynamically[26]. In addition, it has been demonstrated that the alcohol concentration in sweat has a significant correlation with that in the breath and blood, therefore, real-time measurement of sweat alcohol concentration can be adopted to prevent alcoholism[27, 28]. In summary, monitoring human health via wearable swear sensors can not only provide users with a better understanding of their physiological dynamics but also tackle the challenge of clinical resource shortage from centralized and reactive medical care.
Although sweat sensors have been vigorously developed, challenges still exist. To date, most wearable biosensors are prepared on flexible substrates such as plastic thin films and paper. Though such kinds of flexible substrates provide a certain level of bendability, their flexibility/stretchability, as well as air and moisture permeability are far from the requirements of wearing comfort and long-term wearable applications. To tackle these challenges, research has begun on developing wearable biosensors based on textile materials including fibers/yarns and cloths. The properties of textile materials, such as lightweight, intrinsic softness, biocompatibility, promising air/moisture permeability, and high specific surface area, make a significant contribution to the enhancement of the wearability of wearable biosensors. More importantly, biosensors fabricated on textile materials can be seamlessly integrated with clothing via the adoption of conventional textile technologies (e.g. sewing, knitting, and weaving). As such, such a type of textile-based biosensors can be in contact with skin conformably, which can greatly improve the measuring accuracy of target biomarkers without wearable discomfort.
This article mainly aims to provide a comprehensive review of the recent research progress in textile-based sweat sensors as shown in Fig. 1. In Section 2, we briefly introduce the mechanism and the classification of electrochemical sensors by enumerating the representative examples of sweat sensors based on textiles. Section 3 is devoted to state-of-the-art textile-based biosensors. Section 4 introduces three methods to improve the efficiency of sweat collection on textiles. Then, the strategies toward high-performance wearable textile-based biosensors, including the engineering of nano-structured functional materials and system integration strategies, are discussed. Challenges and outlook of wearable textile-based sweat sensors are summarized in the Conclusion Session.
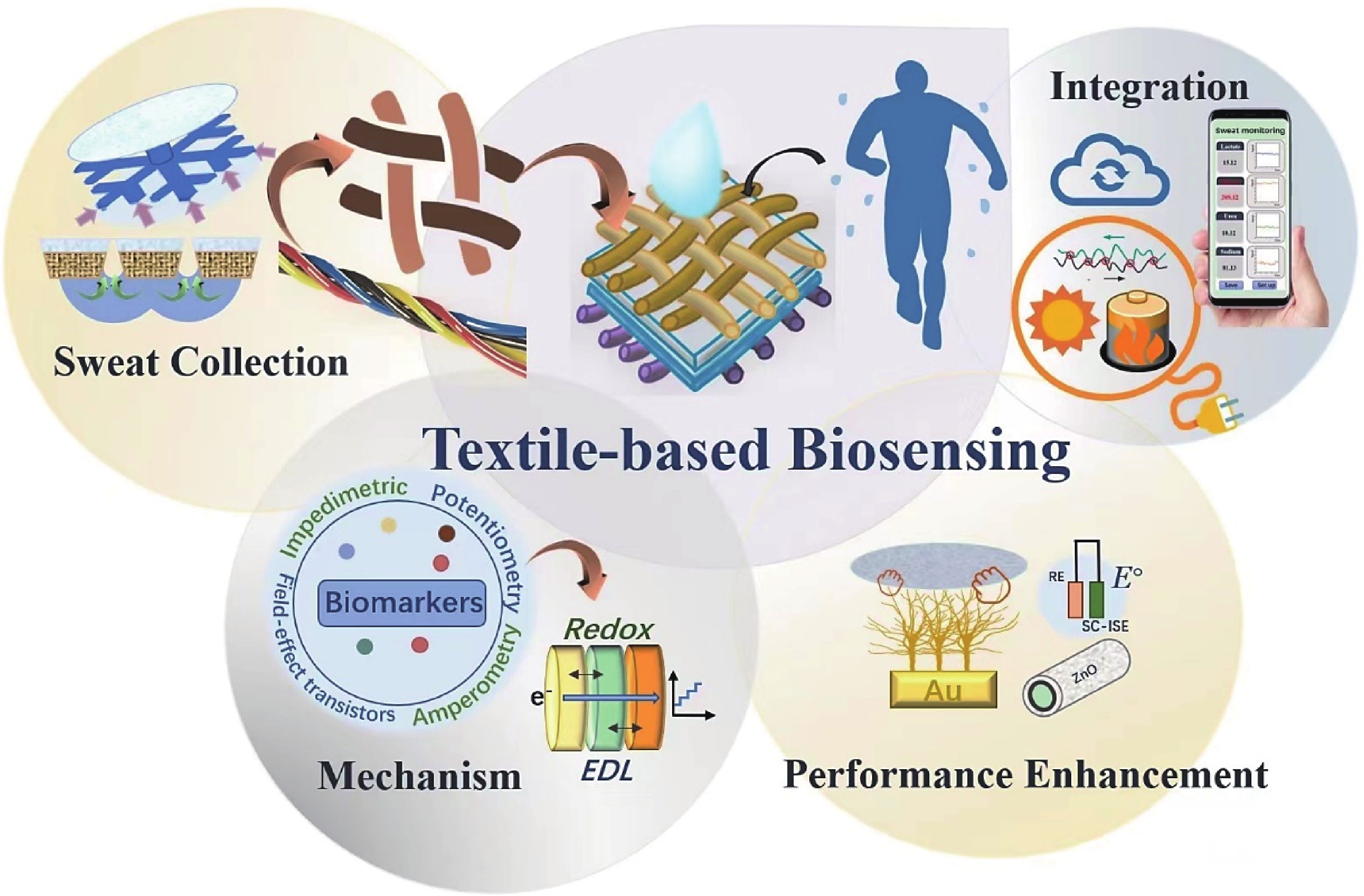
2. Mechanism of biosensors
The most commonly adopted biosensors include colorimetric sensors and electrochemical sensors. Colorimetric sensors can change colors when the target analyte in the to-be-tested liquid binds and chemically reacts with the sensing materials in the sensor electrode. Such type of colorimetric sensor exhibits the advantages of rapid reactions and visible results. However, one major disadvantage of colorimetric detection systems is that they cannot provide continuous tracking of the biomarkers in the sweat.
In contrast, electrochemical sensors can provide continuous and real-time measurement, which is a powerful tool for the monitoring of electrolytes, metabolites, and protein targets. The electrochemical biosensing system generally includes an identification module, a sensor module, and a logistics module (Fig. 2). Identification modules depend on functional bodies that can be specifically recognized by nucleic acids, for example, vectors and enzymes. Sensor modules generally rely on potentiometric (Fig. 2(a)), amperometric (Fig. 2(b)), impedimetric methods (Fig. 2(c)), and field effect transistors (Fig. 2(d))[29]. The data transmission and energy supply modules provide logistical support for the entire system. Textile-based biosensing systems generally use triboelectric generators (Fig. 2(e)), solar cells (Fig. 2(f)), and biofuel cells (Fig. 2(g)) to supply electric energy. Bluetooth, NFC, and other technologies are used to transmit data (Fig. 2(h)).
2.1 Potentiometric biosensors
Potentiometric biosensors convert biomarkers detection into potential signals for sensing[30-32]. Ion-selective electrode (ISE) has been a common choice for the potentiometric analysis for more than 500 years. An ISE typically includes a working electrode (WE) that is coated with an ion-selective membrane (ISM) and a reference electrode (RE), which is used under the near-zero current conditions. The ISM on its WE usually posses two functions: 1) reception of target analyte through the ion exchange or electron transfer concerning the concentration of the target analyte, and 2) signal transduction to convert biological responses into detectable signals (e.g., currents and potentials). When the specific ions are bonded and carried along the membrane, induced ionic activity changes the specific potentials. This static interface sensing method is used to measure the electromotive force (EMF). The measured EMF is directly dependent on the analyte concentration rather than a fixed known concentration, which is based on the Nernst equation[33]:
E=E0+RTnFln[RED][OX], |
(1) |
where E is the cell potential, E0 is the standard potential for the half-reaction, R is the universal gas constant, T is the temperature, n is the number of electrons participating in the half-reaction, F is the Faraday constant, [RED] is the activity of the reducing species, and [OX] is the activity of oxidizing substances. The Nernst factor, RT/F, is temperature-dependent and has a value of 59.16 mV per unit charge for a ten-year change in target analyte concentration at 25 °C[34]. Based on this Nernst equalition, the analyte information can be directly obtained through the substration of the protention of the working electrode by the potention of the reference electrode.
The functionality of textile-based sweat sensors follows the same mechanism as conventional liquid-contacting ISEs. When the sweat reaches the ion-selective membrane interface of the potassium ion sensor, the ionophores in the ion-selective membrane specifically complexe with the absorbed potassium ions. The ionophores then transport electrons to the conductive substrate by two mechanisms respectively, namely the capacitive redox reaction and the EDL capacitance effects[35-37]. Solid-contact materials (SC), which are electrical and ionic conductivity, reveal highly reversible redox behaviors and can be efficiently attached to the surface of substrate electrodes, such as poly(3,4-ethylene dioxythiophene) redox conducting polymers. Target ion concentrations are converted into electronic signals through oxidation or reduction reactions (Fig. 3(a)). Alternatively, ion-to-electron conduction can be formed by the EDL between the ISM and SC interfaces (Fig. 3(b)). It can be considered as an asymmetric capacitor, formed by electrons (or holes) on one side and balanced by ISM ions (cations or anions) on the other. The interface potential depends on the charge volume and the EDL capacitance. As the number of electrons increases, an electrical potential difference occurs. The potential variation mainly depends on the interface between the ion-selective membrane and the aqueous solution with analyte contact. Meanwhile, the interfacial potentials on the reference electrodes remain unchanged. Thus, the resulted open circuit potential between the ion sensing working electrode and reference electrode is correlated with the targeted ion concentrations, and can be decoded by referring to the Nernst equation.
2.2 Amperometric biosensors
Amperometric biosensors, also named as voltammetric biosensors, can detect electroactive substances in biological samples by detecting the potential current relationship. In the amperometric biosensor, the applied potential is variable and can operate in linear or cyclic voltammetric mode. The target analyte can be identified by peak potential and its concentration can be determined by peak current. Unlike the potentiometric biosensor, amperometric biosensor corelates the measured current to the concentration of a specific analyte at a fixed potential.
Enzymatic sensors are one of the most common types of amperometric sensors. Metabolites, hormones, or drugs can be detected by targeting specific enzymes attached to the working electrode. Among various wearable biosensors, glucose sweat sensors, have attracted widespread attention due to their ability to monitor blood sugar as a key indicator of diabetes health management. In previous studies, direct detection of glucose overpotential was high, but it also promoted side reactions (such as oxidation of ascorbic acid, acetaminophen, uric acid, and lactic acid), which hindered sensor selectivity and sensitivity.
2.3 Field-effect transistor biosensors
In the case of the ion-sensitive field effect transistor (ISFET), the ion-selective film is applied directly to the insulating gate of the field effect transistor (Fig. 2(c)). ISFET can also be used to determine the corresponding ion concentration. When such ISFETs are combined with biocatalytic or biocomplex layers, they become biosensors, commonly known as enzymes or immune field effect transistors. Unlike the potential and current sensors of electrode sensors, grid oxide layers play a major role in the ISFET sensors. ISFET's response allows it to be a mains voltage or leakage current by fixing one power source and measuring another.
2.4 Impedimetric biosensors
Impedance biosensors measure changes in electrical impedance. Such changes are usually caused by changes in capacitance and/or resistance of the bio-interface properties of biometric events. When a small sinusoidal excitation voltage (or current) of a certain frequency range is applied, the current (or voltage) generated is measured in an impedance biosensor. It can therefore report biometric events from the phase and/or amplitude changes obtained. An important advantage of impedance biosensors over potentiometric and amperometric biosensors is that they do not damage or interfere with most biometric events, as the applied sinusoidal stimulus voltage is typically 5–10 mV amplitude. There have been several studies to measure blood sugar concentration.
3. The-state-of-the-art textile-based biosensors
We have already mentioned the importance and advantages of fabric as a substrate in the first part. Textiles have become an ideal base for wearable and portable devices due to their lightweight, flexibility, high specific surface area, and easy integration with fabric systems. In terms of dimensions, textiles can be classified into two types of formats, namely one-dimensional (1D) fiber/yarn, and two-dimensional (2D) flat cloths. Fibers are substances composed of continuous or discontinuous filaments with an aspect ratio greater than 50 and a diameter usually ranging from several hundred nanometers to several micrometers. Cloth is made from yarns consisting of fibers and fabricated through different fabric technologies. Textiles have a porosity of up to 99% and provide the advantages of breathability, softness, and stretchability[38], making them as one of the most popular substrates in the field of wearable biosensors. Table 1 summarizes advances in textile-based biosensors with attractive properties for biomarkers analysis.
Classification | Substrate | Fabrication method | Conductivity | Sensitivity | Biomarker | Long-term stability | Reference |
Fiber | Carbon fibers | Integrated TiO2 nanotubes into the conductive carbon yarns (CCY) | High electrical conductivity: CCY has high electrical conductivity. | Desirable sensitivity, wide-range response (10 fg to 1 µg/mL), and good limit of detection (6 fg/mL) | Cortisol | Initial current response remained at 94.70 % after 4 weeks | [49] |
Gold fiber | Prussian blue and glucose oxidase | High electrical conductivity: The average conductivity of the stretchable Au fibers glucose is about 93 S/cm, it has chemical inertness and high conductivity. | A linear range of 0–500 µM and a sensitivity of 11.7 µA/(mM·cm2) | Glucose | Stable chronoamperometric responses in 6 h operation and 8 days of storage | [50] | |
Gold fiber | Dry spinning | High electrical conductivity: superior performance in conductivity | 19.13 µA/(mM·cm2) in PBS and 14.6 µA/(mM·cm2) in artificial sweat | Lactate | 88% current retention after 100 stretching cycles; 71% redox current retention after a 6-day storage | [44] | |
Cloth | Carbon textile | Digital laser writing | High electrical conductivity: CCY has high electrical conductivity. | Desirable sensitivity, wide-range response (10 fg to 1 µg/mL), and good limit of detection (6 fg/mL), along with accuracy. | Glucose, lactate acid, AA, UA, Na+, and K+ | Negligible changes over 4 weeks (<6.4 %) | [51] |
Cloth | Screen-printing and coating | High electrical conductivity: the MWCNTs were an excellent conductive material and could facilitate the electron transfer rate | Acceptable detection range (0.05−1 mM) and sensitivity (105.93 µA/(mM·cm2)) | Glucose | Good long-term stability | [52] | |
Graphene-based nanocomposite | Counter electrodes (CE) filled with modified G-PU-RGO-PB paste | High electrical conductivity: functionalize graphene oxide (GO) using TEPA by solution mixing method to enhance electrical conductivity | The RSD is 3.06%. It can potentially be utilized in detecting lactate from sweat with LOD of 0.4 mM and LOQ of 1.3 mM reliably. | Lactate | The change in anodic and the cathodic current was very negligible after washing. | [46] |
3.1 One-dimensional (1D) fiber conductors
Generally, a strand of fiber loaded with conductive compounds can make up a 1D fiber conductor, which not only has the advantage of air permeability but also can adapt to frequent deformation. In addition, it has good mechanical flexibility and good conductivity[39]. Typically, 1D fiber conductors were fabricated by either coating conductive materials on the surface of insulating fibers or embedding conductive materials into the fiber matrix. For example, to develop super elastic conductive fibers with improved properties and functions, Liu et al. prepared stretchable conductive fibers with a maximum strain of up to 1320% by wrapping specially oriented carbon nanotube sheets around rubber fibers[40]. And this conductive fiber has a resistance change of less than 5% when stretched by 1000%. In addition, it can be used to prepare a strain sensor with a capacitance change of 860%. It is worth noting that due to the 1D structure, these sufficiently long fiber conductors can be directly integrated into existing clothes or fabrics through sewing and other methods while maintaining the original characteristics of air permeability and stretchability[41-43]. Fig. 4(a) is the schematic diagram of the working electrode of the lactic acid sensor prepared with different coatings. 100% sensitivity can be maintained under high tension[44].
3.2 Two-dimensional (2D) cloth conductors
When the 1D fiber wires are staggered according to a certain method, a 2D textile conductor can be further formed. Compared with 1D fiber conductors, 2D cloth conductors have the advantages of being breathable and soft, and also have the advantages of preparing electrical functional modules. Zhao et al. first prepared polyacrylonitrile yarns with strong adhesion of Cu coating and yarns coated with a layer of parylene, and prepared yarns with excellent air permeability by stitching, weaving, and knitting. And found that the material, preparation method, environment, etc. would affect the performance of the obtained sensor. In addition, further weaving has been demonstrated[45], for example, Fig. 4(b) shows a three-electrode system prepared on cotton fabric, which can be used to measure the concentration of lactic acid in sweat and has the characteristics of machine washability[46]. It can be integrated with other electronic systems, including power supplies, etc., which can further realize the complete system on the fabric. This has broad application prospects in the fields of medical care and communication in current society.
In addition to integrating 1D fiber or 2D cloth conductors into the fabric by stitching, weaving, etc., textile-based electrodes can also be directly prepared into conductors through photolithography and screen printing, and other methods to get different shapes of conductors on the fabric[47, 48]. There are many ways to obtain nanoscale copper on the surface of fabrics, and chemical deposition is one of the simple and convenient methods. Lin et al. used this method to pre-decorate the polymerized dopamine layer on the cellulose surface, and then further obtain uniform nano-copper through a Pd2+ catalyst[53]. After experimental tests, the components prepared with this electronic textile conductor showed excellent chemical stability, resistance to washing, resistance to mechanical wear, and the like. This means that 3D-based monolithic fabrics can be effectively integrated with existing garments while ensuring the need for large areas of stretch, breathability, and minimal discomfort.
4. Sampling in wearable sweat sensors
Compared with saliva and tears, chemical indicators in sweat are more suitable for non-invasive health monitoring. This is because sweat is easy to collect, widely distributed, and rich in biomarkers. As such, sweat monitoring has become one of the trends in the research of "personalized medicine" (body fluid diagnosis) in recent years[54-56]. With the advent of wearable electronics technology, vitro diagnostics industry will see explosive growth in the future. Nevertheless, the introduction of new technologies has not yet met the expectations of the rapidly growing medical, consumer, and industrial markets. This is because the amount of sweat that is available for detection is insufficient, while the new sweat will be contaminated by the used sweat. The current state-of-the-art sweat sensors still suffer from low effectiveness for sweat collection, thus failing to make full use of sweat samples. In addition, there is a hysteresis in the concentration change of the biomarker between the sweat and the blood, which usually leads to a failure in the real-time indication of the biomarker in the blood. Therefore, in-depth research on the effective collection of sweat is urgently needed.
Sweat rates, indicating the amount of sweat that is discharged from the human skin within a specific period of time, exhibit huge differences among individuals and even among different regions of an individual. Human bodies sweat all the time, therefore, invisible sweat rate (inSSR) and overt sweat rate (SSR) are two indicators that have been applied to evaluate the sweating level of human under different conditions. Under the resting condition, human bodies also sweat insensibly (inSSR). InSSR is largely determined by the integrity of the skin barrier function that is related to some physiological parameters such as cuticle thickness and blood lipid composition. Usually, skin with thicker stratum corneum and lower blood lipid composition could exhibit higher inSSR[57]. As such, inSSR varies from different regions of the body: the hands and feet normally lost the largest amount of sweat (45–90 g/(m2·h)), while the head and neck lost a less amount (20–40 g/(m2·h))[58]. Different parts and body states of SSR are also different. The specific detailed topographic map of SSR is shown in Fig. 5(a). Sweating rates are not only affected by gender, weight, and age of the human body but also largely affected by some external factors associated with living environments (e.g., space, wind speed, intensity of solar radiation, etc.).
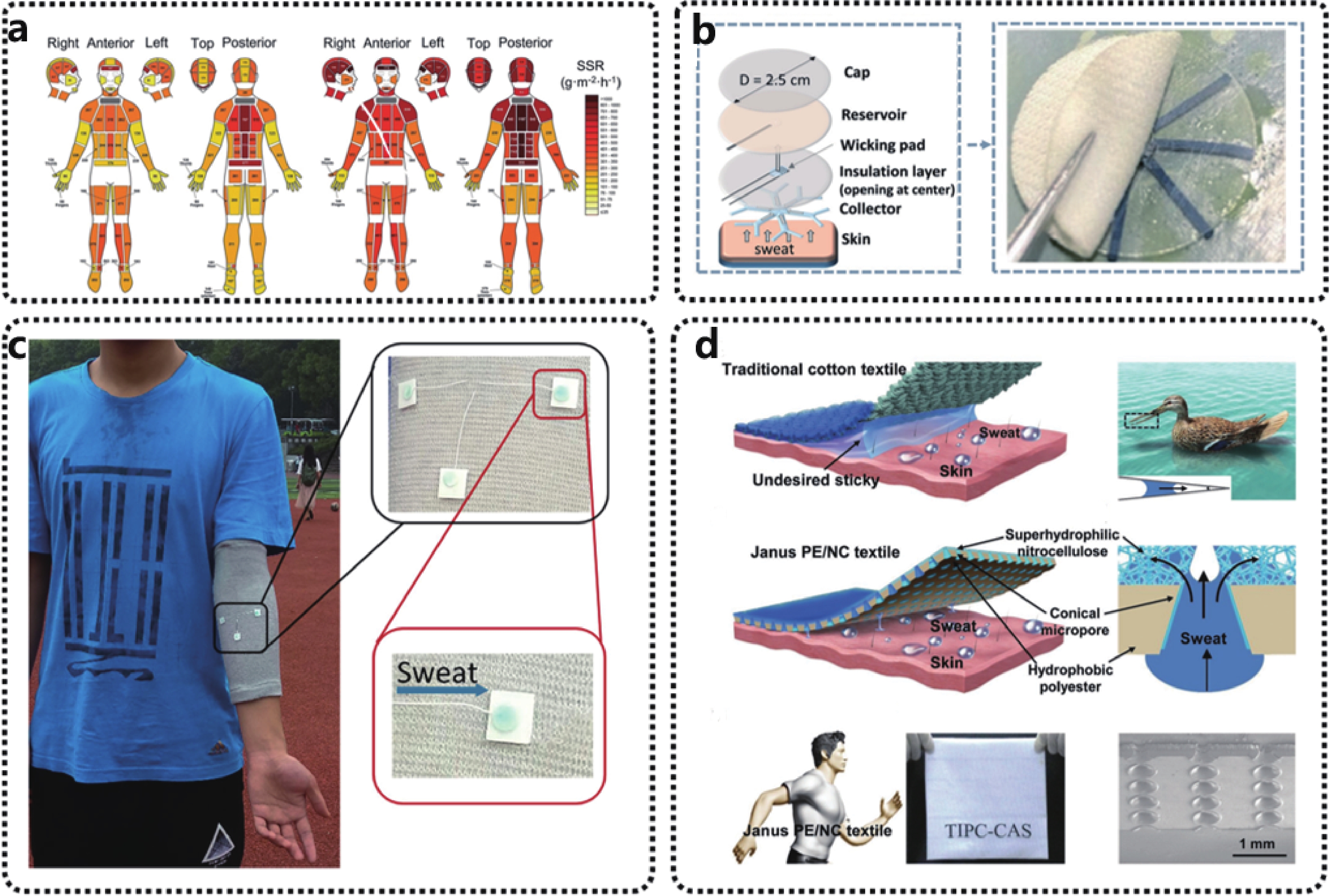
Traditional sweat collection methods include local sweat collection with epidermal patches[59], polymer bags[60], microcatheters[61], and whole-body irrigation techniques[62]. However, the subsequent analysis is normally carried out separately, which may lead to critical issues such as sweat evaporation, chemical degradation, and/or contamination of the sweat samples. These cannot meet the requirements of dynamic, real-time, and accurate sweat analysis. Therefore, researchers are constantly developing and optimizing new sampling methods, to achieve autonomous and continuous sweat sensing with emerging wearable and skin-compatible devices[63-65]. Currently, new sweat sampling technologies in wearable sensors mainly include designing sweat channels, adopting functional water-absorbing materials, and developing novel textiles with superhydrophobic/superhydrophilic Janus structures.
The earliest successful research on new sweat collection technology was in 2016 when Liu et al. first created a sweat guide channel to effectively collect sweat[66]. Subsequently, textiles with rapid water absorption ability were designed with branch-patterned microchannels, which could achieve sweat collection (Fig. 5(b))[67]. The optimized microchannels had a tree-like geometry designed to eliminate sweat spillage. Efficient sweat collection is achieved through a shorter induction time (<1 min after sweating) and a maximum sweat flux of up to 4.0 µL/cm.
Textile embedded with tapered micropores, which has a hydrophilic inner surface (Fig. 5(c)), has been developed for directional liquid transport (1246% ultra-high directional water transport ability). In addition, by absorbing sweat with the functional absorbent patch, and transporting sweat through the hydrophilic cotton thread, the sweat collection efficiency has been greatly improved. This enables low-cost sweat collection[68]. However, the physiological comfort of the functional water-absorbing materials that are applied for sweat collection are poor, because the collected sweat within the hydrophilic textiles cannot be evaporated. To tackle this challenge, Dai et al. designed a Janus textile. When a liquid got in contact with the superhydrophilic carbonized cellulose layer of the Janus textile, the liquid could be pumped to the hydrophobic polyester layer through a capillary-driven hydrophilic conical micropore (Fig. 5(d))[69].
5. Strategies toward high-performance wearable textile-based biosensors
Though many studies have made good progress in the field of wearable biosensors, there is still room for the performance enhancement of sensors. For example, the previous textile-based sensors have limited anti-interference ability and low sensitivity. Moreover, how to integrate Bluetooth, power supply, and other modules with sensor modules to obtain a complete system that can transmit signals and be self-powered is also a big challenge. Of course, the first thing that needs to be done is to connect the different modules in a stable manner. This chapter explores some strategies for improving the performance, connection methods and integrity of textile-based wearable biosensors on these three points.
5.1 Nano-structured functional material for performance improvement
The flexible ion sensors based on human metabolites have the advantages of non-invasive acquisition, fast update speed, and abundant types of factors to be analyzed in the acquisition of liquid to be measured. Long-term human metabolite monitoring can be matched with human health status. However, the current flexible sensors have the problems of insufficient stability and short lifespan to support long-term operations, due to the drift of the output signal, poor long-term stability, and poor preparation repeatability of these types of sensors. A wearable sensor that can continuously measure Ca+ and pH in body fluids was fabricated in 2016[71], but it has poor wearing comfort and repeatability. In 2019, an integrated wearable sensor was developed[72], which exhibited the advantages of being small and lightweight, and could measure Ca+ and NH4+ ions at the same time. However, the sensor took a long time for stabilizing the output signal, and the signal drift value is large. A highly stretchable, strain-insensitive, non-invasive human lactate sensor was prepared in 2021[44], but there is a lack of cycle test data in the work so that the long-term stability of the as-made sensors remained unknown. Researches especially on the signal drift mechanism, long-term stability, and repeatability of such types of sensors are highly required.
Potential electrode drift, E° drift, is associated with inherent sensor defects, including slow reaction equilibrium and polarization interfaces. Besides, disturbances namely light, oxygen, and carbon dioxide can lead to the reaction instability of all-solid electrodes[35]. Ideally, solid contact should be an unpolarized interface with a high exchange current density and unaffected by small input currents. However, virtually all solid contacts are polarized to some extent. Electrode potential can be further stabilized by designing solid contacts with high redox or double high redox large double-layer capacitance, which could be realized by introducing nano-structured materials such as metal nanoparticles, carbon nanotubes, and other nanomaterials[73-75]. To solve the above problem of signal drift and expand the electrochemical sensor strength of biosensors simultaneously, Li et al. incorporated gold dendritic nanostructures on the electrodes, and significantly improved the detection limit of sweat sensors for biomarkers through integrated surface innovation at the physical and chemical levels (Fig. 6(b))[76]. The gold nano dendritic layer obtained by potentiometric deposition has a greatly increased specific surface area compared to others, which can improve the sensitivity of the device. In addition, the dendrites can provide sufficient attachment cross-sections for enzymes and other key molecules, thereby enhancing the performance of the sensor. In these works, an improvement in the detection limit of levodopa, a special detection substance in sweat, was achieved, down to about 1 µM.
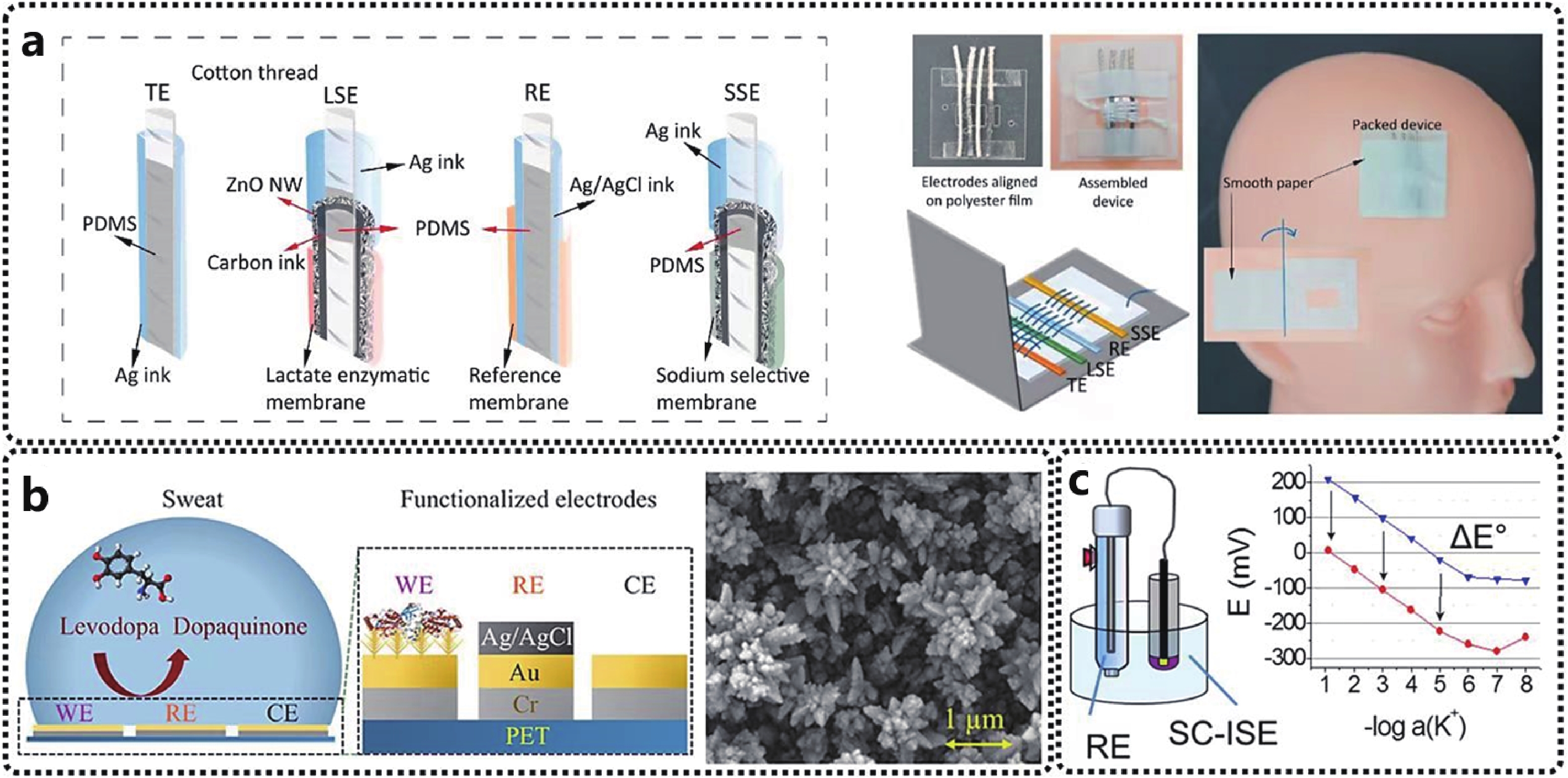
To enhance the long-term stability and sensitivity, Zhao et al., taking advantage of the excellent physical and chemical properties of semiconductor ZnO nanowires, such as good biocompatibility, high surface electron transfer rate, and simple preparation process, proposed a modified ZnO nanowire-based on the excellent physical and chemical properties (Fig. 6(a)). A novel sweat sensor[77] with a conductive thread, improves the sensitivity of the device and confirms stable signal test results even during body movement.
Apart from drift and stability, uniformity of the initial voltage consistency is also an important paratmeter. As mentioned above, textile-based sweat sensors have facilitated the development of clinical analysis toward portable nursing and home diagnostics, to make convenient and accurate health monitoring devices accessible to the general public. However, the existing situation is that there is a drift of the sensor output signal from the device to the device, and even between different tests. For this reason, the calibration of the system requires a series of expensive and complex calibration steps, which is impractical. This means that the initial voltage is different for each test, which is a critical issue that needs to be addressed. Wanamo et al. found an easy and no external instrumentation method, which can control the standard potential (Fig. 6(c))[78]. The specific operation method is to use a metal wire to short-circuit the WE and a commercial RE. Because commercial electrodes are filled with solution and redox capacitors, corresponding to the case of supplying 0 V power to the working electrode, the reduction/oxidation drive of the conductive polymer film is optimized by short-circuiting the working electrode, thereby the standard potential of the electrode will be changed. Experiments show that when the WE short-circuit the commercial RE, the PEDOT:PSS layer is reduced so that the potential of the WE is close to the potential of the RE, and the adjustment of the standard potential is achieved when the potential difference is close to zero.
5.2 Integration strategies for textile-based sensing systems
In addition to the improved performance of individual sensors, integration with other components is also important for practical applications. An ideal textile-based sweat sensor test system needs to include test modules, power supply, signal processing modules, etc. In this regard, the performance of sweat biosensors has been refined. In addition to this, much research has been done on the integration of self-powered sensor systems or energy storage devices at this stage. The idea of integrating sensor systems with wireless communication components has also been investigated. In this section, integration strategies for textile-based sweat sensors will be introduced, including, energy supply system integration and wireless communication module integration.
Most of the biosensors can be prepared by electrochemical deposition, spin coating, drop coating, preparation, etc. The sensors prepared in this way have good adhesion to the original textile substrate with certain roughness. Phenomena such as delamination do not easily occur. Therefore, in order to well connect the sensor of the fabric substrate to the circuit, it is only necessary to ensure that the conductive material of the sensor substrate is well connected to the other components. In general, in order to implement a sensing system, the sensing module also needs to be connected to the transmission module, the signal processing module and the power supply module. Typically, mechanical, physical, and chemical methods are normally adopted for connecting and bonding different modules and devices. Smaller components can be inserted directly into a 2D conductive cloth. They can also be connected first to 1D conductive fibers by soldering and bonding plating physical and then integrated into the garment by weaving and other methods. In addition to this, they can be connected to individual platforms, such as snap fasteners, before being integrated into textile systems. Chemical methods utilizing covalent bonding and plasma treatment also contribute to robust interconnection. Physical bonding has been the preferred choice of most people due to its convenience and solidity point.
The soldering method can be used when the components to be connected have the electrical conductivity of metal and are resistant to high temperatures. An example of using this method is shown in Fig. 7(a). Electronic components are soldered to metal leads and connected to the circuit inside the garment[79]. Although this method can provide good electrical conductivity, it has certain disadvantages. First, the materials used are restricted. Second, the soldered circuit is rigid and can cause discomfort to the wearer and limit body movement.
For flexible system components with certain deformability, their direct connection is generally through traditional wire/conductive tape[80, 81], flexible solder/conductive paste[82, 83], or stretchable conductors. Snap fasteners, the most widely used connector in textile-based systems today, offer several advantages. First, it can be removed and reused in facile manner. Secondly, the wires are connected to the pin of the electronic components, and the other end are connected to the snap, which is then sewn to the garment as a whole shown in Fig. 7 (b)[84]. Such snap fasteners modules offer the merits of softness and bendability. Such fabric system components, because they can be assembled with the clothing through sewing and other lamination techniques, the system integration is more designable and convenient and can largely retain the original breathability, stretchability, and comfort.
Although the snap connection method is very popular, frequent disassembly and installation can wear out the snap fasteners. It requires further evaluation on their mechanical stability and robustness. Besides, there could be side effect on signal transmission. With the active development of textile-based sweat sensor systems, it becomes challenging to provide a stable, efficient power supply for such systems over the long term. In addition to the high energy requirements, there is also a strong need for compact and flexible energy harvesting and storage devices that do not compromise the convenience and comfort of flexible sweat sensor systems. Self-driving sensors integrated with external sources such as mechanical, thermal, and nanogenerators[85, 86], solar energy[87, 88] as well as near-field wireless power sources have been extensively explored[89-93]. In this regard, wireless connections, such as the development of NFC transmission systems, are preferred in wearable electronics.
Wireless technology can connect MUC with wearable sensors without physical limitations, which can provide real-time health monitoring and clinical notification. Compared with wired interconnects, wireless technologies require a more separate power supply for each sensor, by rigid batteries or cumbersome energy harvesters, which leads to skin conformability and user comfort and energy supply modules that need to be replaced regularly. In addition, encryption is needed as well to protect data transmissions from eavesdropping. Near-field communication (NFC) is a better candidate for wireless technology powered by the sensor of a wireless reader[94-96]. Battery-free, low-cost, and resistant to eavesdropping NFC-based sensors have become a popular choice for integration with electronic devices. Lin et al. create body-used wireless power and data connections using close-field assistive clothing (Fig. 8(a))[97]. These garments connected low-cost conductive threads and computer-controlled embroidery to proximity functional textile patterns and created a battery-free sensor network. These close-field garments are entirely textile-based and suitable for everyday wear. They used NFC to develop textile designs without any modifications and demonstrated their applications in monitoring spinal posture using multiple wirelesses, battery-free sensors and continuously measuring temperature and gait during exercise.
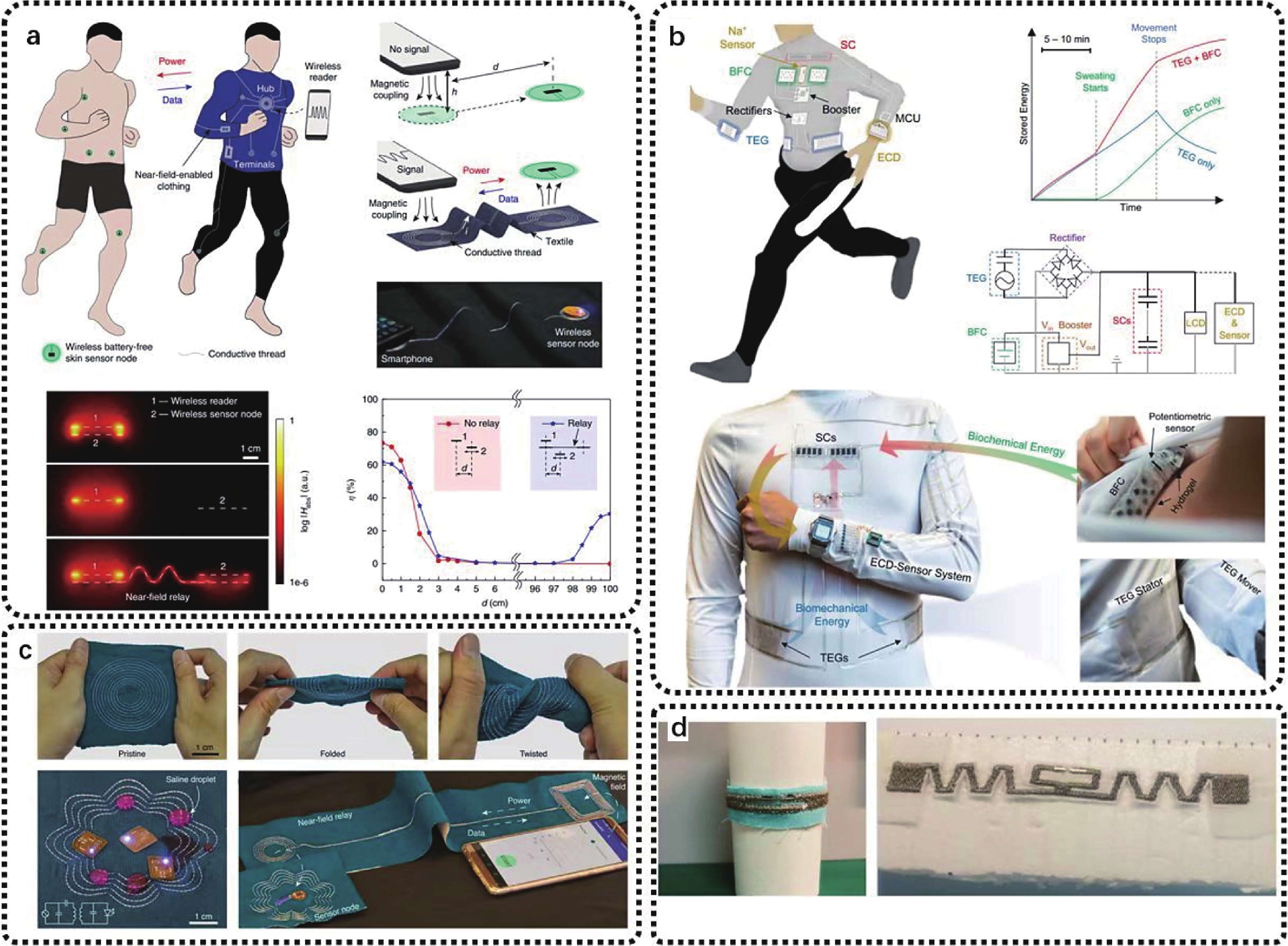
In addition to the near-field wireless power supply and data transmission, wearable sensors are also integrated with energy modules. The most commonly used energy harvesting systems on fabrics include solar cells and triboelectric generators. The integration with this module can meet the large energy demand of the sensing system, replacing the troublesome wired power transmission for self-supply[101]. Triboelectric generators provide an alternative to piezoelectric and can be used to capture mechanical kinetic energy compatible with skin mounting systems. The device generates an electrical charge through contact and separation between two surfaces with different charge polarity, i.e., charged and electrostatic induction[102]. The charge separation between the two surfaces creates a voltage difference[103]. Triboelectric generators (TEGs) mainly operate in one of two basic operating modes: contact mode and sliding mode. Lu et al. fabricated a textile-based microgrid (Fig. 8(b))[95]. The system relies entirely on human activity to coordinate its work. With an energy budget, the TEGs can continuously and efficiently power a liquid crystal display (LCD) or sweat sensor-electrochromic display system during a pulse session, and a 10-min workout halves startup time and triples run time. The flexible textile-based bioenergy microgrids realize the design principle of the compatible form factor, corresponding performance, and complementary functions, offering attractive prospects for designing and operating efficient, sustainable, and autonomous wearable systems. There are other examples of system integration on textiles. For example, liquid metal fiber could be embroidered onto cloth for the establishment of a wireless connection with wearables and implants nearby (Fig. 8(c))[96]. As shown in Fig. 8(d), there have also been studies of embroidered textile-based antennas on textiles[97], which show great potential in wearable applications.
6. Challenges and perspectives
This paper reviews the recent progress of wearable sweat biosensors developed on the basis of textiles, especially on their working mechanism, classification, fabrication methods, and the strategies for performance improvement and system integration. The non-invasive electrochemical sweat biosensor is capable of detecting a range of analytes, including electrolytes, metabolites, drugs, hormones, and more. What’s more, the methods of sweat collection were analyzed. This paper also covers the progress of the integration of self-powered modules and wireless signal transmission modules. The design of energy harvesters and signal transmission modules with high stretchability and flexibility has been a research focus in the past decade. In the field of novel nanomaterials, material advancements in terms of flexibility and stretchability to improve user comfort are underway. Strategies are being proposed to improve dynamic performance, including material selection and structure fabrication, leading to promising systems with high performance. The rapid development of today's ion electrochemical sensors not only broadens the application scope of electrochemical analysis, but also significantly improves its sensitivity detection capability, rapid online analysis, and microanalysis. Textile-based sweat sensors can not only utilize nanostructures to improve sensitivity but also combine biomolecular responses to improve selectivity. The electron transport capability at the interface by increasing the ion exchange reaction and modification materials. Therefore, with the continuous improvement of wearable ion sensors, their application fields will be more extensive, involving the determination of inorganic, organic, and biological and their activity. However, the reusability, washability, long-term stability, and fabrication repeatability of textile-based sweat sensors still need to be further studied. It is worth mentioning that the sweat collection strategy deserves the attention of researchers while other parts of the technology are still well developed, and there are still several challenges for real-time, non-invasive sweat monitoring in future applications.
The first critical challenge should be how to effectively collect sweat from human skin. Currently, high-intensity exercise or iontophoresis is the main method to collect sweat, however, even during high-intensity exercise, the sweat secretion rate does not exceed 20 nL/min/gland[104]. More importantly, studies have shown that some electrolyte concentrations in sweat collected after exercise do not reflect the corresponding plasma electrolyte concentrations[105]. Stimulation of local sweating by iontophoresis is more suitable for the disabled, the elderly, or infants, but the intensity of use of current devices must be carefully controlled. This is because repeated application of iontophoresis at the same location may injure the skin[106]. Moreover, iontophoresis is the only one-time sampling and sensing and does not exploit the potential advantages of sweat collection (continuous and repetitive)[107].
Another challenge lies in the mixture of new sweat and used sweat, as well as the potential contamination of the tested area. Once sweating glands secrete and sweat comes into contact with the skin, it can be difficult to remove contamination from the surface or surrounding environment, which can have a great impact on the accuracy of sensor readings. Simple cleaning of the skin surface does not alleviate contamination such as bacteria, which are estimated to be as high as 1010 cm-2 on the skin, and consumes glucose, proteins, and cellular metabolites in sweat, potentially leading to considerable concentration errors[108]. A possible solution is to isolate the sweat from the skin surface by applying a layer of oil (such as petroleum jelly) to the skin surface[109], but such an approach would have adverse effects on wearable sweat sensor detection.
The third challenge is the unknown concentration caused by the filtration and dilution of biological macromolecules (such as proteins). Large analytes like proteins are filtered in sweat, which means they are highly diluted compared to blood concentrations[110]. At the same time, the protein ratio between blood and sweat is correlated[111]. If the molar concentration of protein in blood is nM-pM, the relative protein concentration in sweat will be diluted to pM–fM, a very different concentration to measure. But there are a few methods that successfully detect proteins in sweat. One is microfluidic monitoring, where the concentrations of many proteins change slowly over several hours, so less sampling is required to lay the foundation for accurate sample detection[112]. The second is protein concentration, which can be achieved by simple selective membrane technology, allowing more time for the protein to enter the sweat, thereby improving the overall concentration and measurement accuracy. Moreover, the concentrations of some analytes represent only the skin or the eccrine glands themselves and have no clinical significance. For example, sodium chloride excreted during exercise has no serum correlation[104]. Another example is lactic acid, the by-product of local metabolism during sweat production. However, it does require specific analytes or very low sweat production rates to obtain meaningful metabolite profiling.
The final challenge concerns the washability of the system. Since the sensor needs to be in contact with the sweat before biomarkers, contamination is unavoidable. To save the costs, it is usually possible to remove the remains and residues washing. However, due to the soft texture of the selective membrane, the cleaning step can damage the surface or even the inner structure with intense cleaning. This can result in the degradation of the sensor performance or even the destruction of the sensor. Surface modification with biomimetic micro/nano structures to achieve self-cleaning effect would be one of the preferred strategies. Besides, materials engineering to realize washable packaging or protective layers is also expected to enable reusable textile smart electronics and systems.
In conclusion, research on textile-based wearable sweat biosensors connects the traditional textile industry with non-invasive human health monitoring and innovative wearable and flexible electronics. Although many notable advances have been made as described in this review, research on wearable sweat sensors is still at an early stage and there are still many challenges to be addressed in the future. Such challenging work requires interdisciplinary teamwork in research communities such as materials, electronics, nanotechnology, and textiles. Shortly, significant progress is expected in developing high-performance and cost-effective electrode materials, high-efficiency sweat harvesting strategies, new packaging strategies, and advanced device structures for high-performance and affordable wearable sweat sensors.
Acknowledgements
This work was supported by the National Natural Science Foundation of China (62201243), Fundamental and Applied Research Grant of Guangdong Province (2021A1515110627), Southern University of Science and Technology (Y01796108, Y01796208), RGC Senior Research Fellow Scheme of Hong Kong (SRFS2122-5S04), the Hong Kong Polytechnic University (1-ZVQM), RI-Wear of PolyU (1-CD44), and Shenzhen Science and Technology Innovation Committee (SGDX20210823103403033).