Recently, all-inorganic perovskites have attracted attention due to good thermal stability[1-12]. Among them, CsPbI3 has the most desirable optical bandgap (~1.7 eV) for applications in optoelectronic devices[13-16]. In general, making black-phase CsPbI3 film requires a high-temperature annealing up to 320 °C[17, 18], which inevitably raises energy consumption. Though being made at high temperature, the resulting black-phase (α or β phase) CsPbI3 film still suffers from an undesirable phase transition under ambient conditions[19, 20]. Several strategies have been developed to lower the annealing temperature (90–100 °C)[20-26], it is still challenging to stabilize black-phase CsPbI3 under ambient condition with high humidity and without a tedious annealing process. Herein, we developed a simple crystal redissolution (CR) strategy to make stable black-phase CsPbI3 film in ambient air with high humidity and without post-annealing. 4-N,N-dimethylamino-4ʹ-Nʹ-methyl-stilbazolium tosylate (DAST) can chemically interact with CsPbI3 to reduce the formation energy of black-phase and inhibit CsPbI3 to undergo black-to-yellow phase transition.
Fig. 1(a) shows the CR approach. By using the perovskite precursor consisting of PbI2, CsI and HI, a light-yellow film was obtained in ambient air, which is due to the existence of both yellow-phase δ-CsPbI3 and PbI2, as evidenced in XRD pattern (Fig. 1(b))[22]. In contrast, by using CR-derived perovskite precursor (Fig. S1), a mirror-like black CsPbI3 film was obtained even under 70% relative humidity, which uniformly covered the entire substrate (inset in Fig. 1(c)). Compared with the control sample (Fig. 1(b)), there is no PbI2 signal (12.6°) in XRD pattern (Fig. 1(c))[27], which is due to a more direct conversion and rapid self-assembly from CsPbI3 crystals to CsPbI3 film, rather than the complicated competition among Pb2+, Cs+, I– ions and solvent molecules[27, 28]. The diffraction peaks at 14.98° and 29.20° are the typical (100) and (200) planes of black-phase β-CsPbI3. Meanwhile, the absorbance of the control film sharply declined after 450 nm, while CR-derived black CsPbI3 film presents an absorption onset at 733 nm (Fig. S2), which agrees with the previous report on β-CsPbI3 film[12]. For the control film, inferior surface coverage was observed (Figs. S3(a) and S3(c)). And CR-derived film shows better surface coverage (Figs. S3(b) and S3(d)).
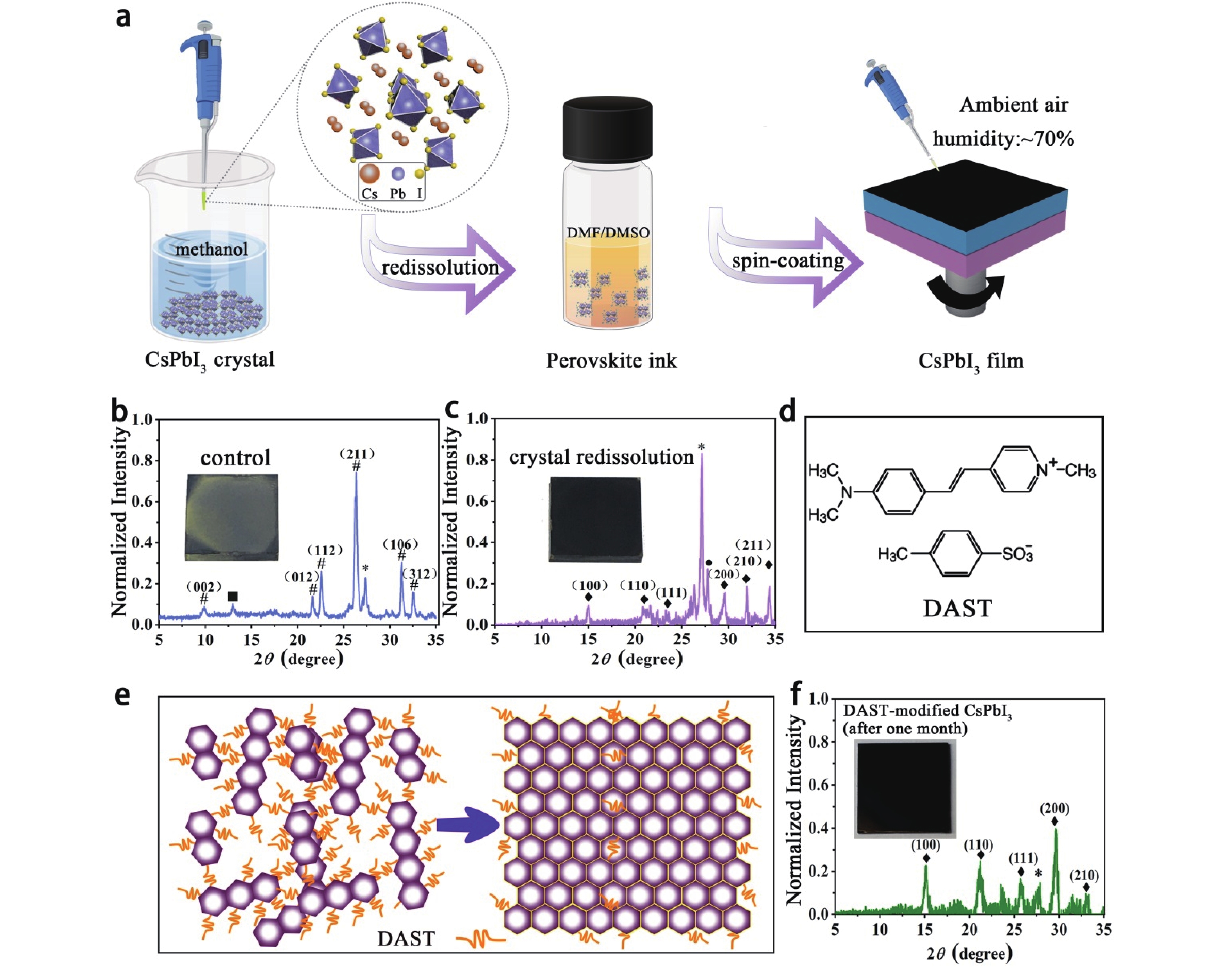
Black-phase CsPbI3 film gradually degraded and underwent phase transition when stored in air for one week, as evidenced by the gradual decrease of absorbance (Fig. S4). To further improve phase stability and optoelectronic properties of β-CsPbI3 film prepared by CR strategy, we introduced the DAST additive (Fig. 1(d)). DAST not only maintains black-phase CsPbI3 structure, but also slightly enhances the crystallinity and promotes the crystal growth orientation along (100) and (200) planes (Fig. S5). DAST also helps to reduce the grain sizes (100–200 nm) and improve the surface coverage of the resultant β-CsPbI3 film (Fig. S6). DAST molecules can interact with CsPbI3 via robust bidentate coordination, thus impeding grain growth due to the steric hindrance effect (Fig. 1(e))[11]. The interaction between DAST molecule and β-CsPbI3 was studied by FTIR (Fig. S7). The pure DAST shows characteristic signals at 1023 and 1666 cm–1, corresponding to C=C bond and benzene group, respectively. DAST-modified CsPbI3 film also shows similar peaks, but with a slight shift, suggesting possible interaction between zwitterion and ions in perovskites[20]. The DAST-modified CsPbI3 film was stored at room temperature in air with a relative humidity of ~35%. There was no obvious degradation observed even after one month, as proved by XRD pattern (Fig. 1(f)).
In short, by using the CR strategy, we successfully stabilized the black-phase CsPbI₃ film in ambient air with >70% humidity. DAST can further stabilize the black phase. The approaches in this work will be useful for developing efficient perovskite solar cells.
Acknowledgements
We appreciate the National Natural Science Foundation of China (22005355) and Guangdong Basic and Applied Basic Research Foundation (2019A1515110770). L. Ding thanks the National Key Research and Development Program of China (2017YFA0206600) and the National Natural Science Foundation of China (51773045, 21772030, 51922032, 21961160720) for financial support.
Appendix A. Supplementary materials
Supplementary materials to this article can be found online at https://doi.org/10.1088/1674-4926/43/3/030501.