Metal halide perovskites have made rapid progress in photonic and optoelectronic applications since the first report of solid-state perovskite solar cells in 2012[1]. Perovskites feature superior luminescence properties beneficial for the application in light emitting diodes (LEDs), such as high photoluminescence quantum yields (PLQYs), narrow emission, and tunable bandgaps[2, 3]. Low-cost perovskite LEDs (PeLEDs) have attracted interests, causing fast enhancement of their performances, and demonstrating great potential in next-generation lighting and display applications.
Quasi-2D perovskites represent an important category of perovskites, with great success in light emission applications due to their unique and excellent optoelectronic properties. It is characterized through a sandwich structure that PbX6 octahedra sheets are packaged by large ammonium cations, forming a layered either Ruddlesden-Popper (RP) phase with formula of L2Sn–1PbnX3n+1, or Dion-Jacobson (DJ) phase with formula of LSn–1PbnX3n+1, where L is monovalent or divalent ammonium cation, S is small cation, X is halide anion, and n is the order of quasi-2D perovskite (the number of stacked PbX6 sheets). Quasi-2D perovskites with reduced dimension can construct self-organized multiple quantum-wells to induce both dielectric- and quantum-confinement effects, thus improving exciton binding energy over several hundred meV and enabling PLQYs up to 100%[4-6]. The emission behavior of quasi-2D perovskites is also determined by exciton recombination kinetics, and the management of singlet and triplet excitons in quasi-2D perovskites is fundamental for designing efficient PeLEDs and laser gain media[7]. In addition, there are efficient energy funnels from low-n value to high-n value domains in mixed-phase quasi-2D perovskite emitting layers, leading to accumulated excitons in the recombination centers, which is beneficial for high radiative recombination efficiency and PLQY even at low pump power densities[8].
Efforts have been devoted to designing and fabricating high-quality quasi-2D perovskite films for laser and LED applications. Qin et al. first reported stable room-temperature continuous photoinduced perovskite laser[9]. Meanwhile, extensive works on quasi-2D perovskites have been performed to improve the performance of PeLEDs, demonstrating highly efficient green devices with over 25% EQE, red and near-infrared devices over 20%, and blue devices over 10%, respectively[10-12]. Domain distribution controlling and defect passivation in quasi-2D perovskite emitting layers are the most effective strategies for quasi-2D PeLEDs.
Quasi-2D perovskite films feature a mixed phase rather than a single phase because the formation energies for different phases are similar. The solubility difference of precursor components and steric hindrance difference between cations cause a wide domain distribution, which may cause several problems. First, low-n value (n = 1 and 2) domains with reduced crystal size accompany with more traps, resulting in serious trap-induced nonradiative recombination. Second, though the energy transfer from low-n domains to adjacent high-n domains is faster than trapping, the energy loss still inevitably exists. At last, the higher-n (n > 10) domains tend to form free carriers and make nonradiative recombination, which also yields modest PLQY. It is important to narrow the distribution to avoid nonradiative recombination.
Zhang et al. made quasi-2D perovskite films with a narrow domain distribution by using two additives, ZrO2 nanoparticles (NPs) and cryptand[13]. ZrO2 NPs promote synchronous crystallization by facilitating the interaction between solvent and antisolvent, and cryptand complexing with Pb2+ retards the crystallization of high-n domains by forming an intermediate state, thus enhancing EQE of green PeLED from 16% to 21%. Narrowing domain distribution can also improve emission color purity and achieve spectra-stable blue devices. Yantara et al. reported that composition engineering through prudent selection of the cations coupled with rapid nucleation can result in a narrow domain distribution[14] (Fig. 1(b)). Methanesulfonate was used to reconstruct quasi-2D perovskite, yielding green PeLEDs with >20% EQE (Fig. 1(c))[15]. Ma et al. used a bifunctional additive, tris(4-fluorophenyl)phosphine oxide (TFPPO), to prepare quasi-2D perovskites with a monodispersed domain distribution[10]. The fluorine atoms make hydrogen bonding with organic cations, controlling their diffusion and suppressing the formation of low-n domains (Fig. 1(d)), and phosphine oxide moiety passivates grain boundaries via coordinating with the unsaturated sites. Green PeLEDs with an EQE of 25.6% were obtained.
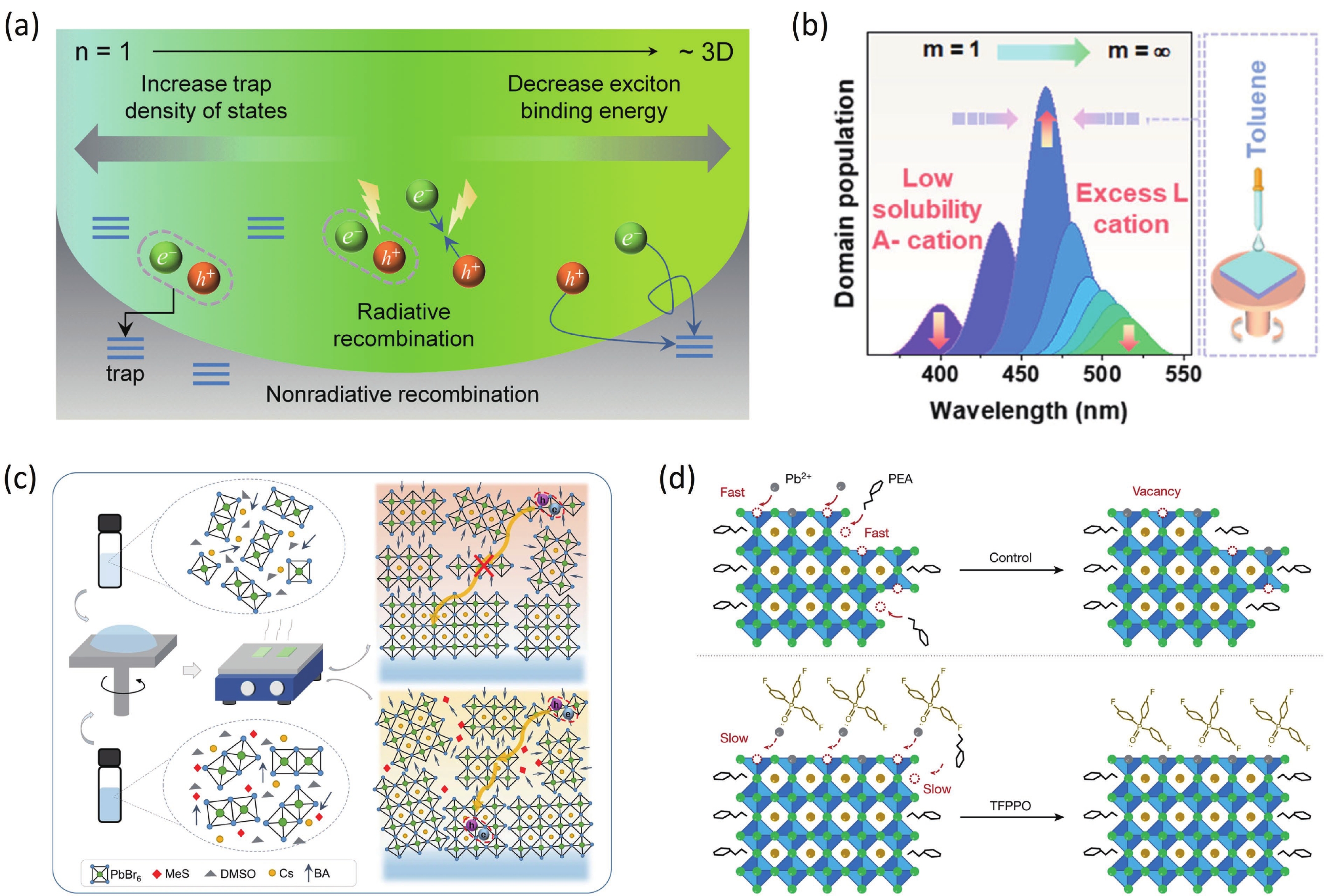
Inevitable defects and traps can easily form in polycrystalline perovskite films during crystallization in solution-processing methods. Compared with 3D perovskites, quasi-2D perovskites with reduced crystal size have higher defect densities. The charged defects can act as nonradiative recombination centers to decrease emission efficiency.
Halide anion vacancies in quasi-2D perovskites are usually shallow-level defects (at least for Br- or I-containing perovskites), which are not detrimental to device performance. Defects with deep trap states such as interstitial or anti-site defects are almost absent in perovskites since they have high formation energies. Coordination-unsaturated Pb ion can act as nonradiative recombination centers, which should be treated seriously during passivation[16, 17]. Understanding the effect of defects on device performance, and developing passivation strategies are critical for enhancing the performance of quasi-2D PeLEDs.
Passivators with X=O bond (X: P, C, S or other atoms) are effective to coordinate with Pb defects. Among them, the P=O:Pb dative bond showed a strong binding energy of 1.1 eV, avoiding nonradiative recombination caused by Pb defects[4]. Passivation agent with P=O such as trioctylphosphine oxide, triphenylphosphine oxide, as well as their derivatives all enhanced PLQY of quasi-2D perovskite films[10]. Meanwhile, Pb defects exposed at the edge of quasi-2D perovskite can also cause the instability. Photogenerated and electrically-injected carriers diffuse to perovskite edges and produce superoxide, causing rapid photodegradation (Fig. 2(a)). Quan et al. reported an edge-stabilization strategy of passivating exposed Pb defects by P=O bonds, which improved EQE and stability of quasi-2D PeLEDs[4]. C=O and C–O–C bonds also demonstrate outstanding Pb-defect passivation ability[6, 18]. Liu et al. developed a dual-additive strategy to prepare quasi-2D perovskite films with low defect density and high environmental stability by using 18-crown-6 and poly(ethylene glycol) methyl ether acrylate (MPEG-MAA) as additives (Fig. 2(b))[18]. The EQE of green PeLEDs reached 28.1%. 1-butyl-3-methylimidazolium tetrafluoroborate (BMIMBF4) ionic liquid can also passivate Pb defects[19]. Spontaneously formed targeted distribution of the ionic additive well matches the defect site distribution (Fig. 2(c)). Novel passivators with more functions have been explored. Phosphonate-triphenylphosphine oxide with dual roles of passivating defects and enhancing carrier radiative recombination boosted EQE over 25%[20]. Phosphine oxide can enhance PLQY by passivating Pb defects, and phosphonate with strong electron affinity can accelerate carrier radiative recombination by capturing injected electrons (Fig. 2(d)). Defect passivation plays critical role to realize highly efficient PeLEDs.
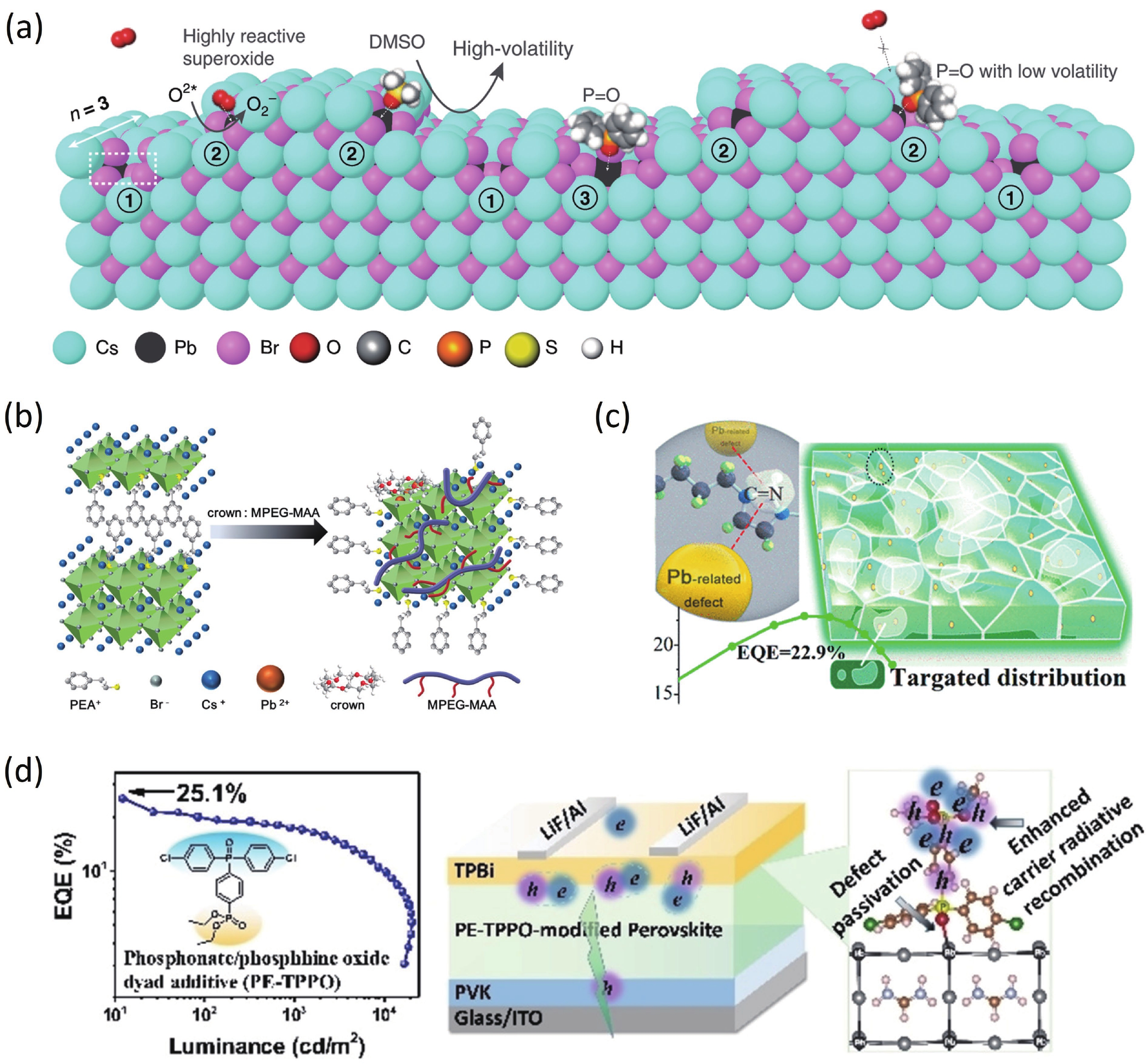
In short, domain controlling and defect passivation are effective approaches to enhance EQE for quasi-2D PeLEDs. There are still challenges, such as highly efficient pure red and blue emission, long-term operation stability, and environmental safety.
Acknowledgements
We thank the National Natural Science Foundation of China (22075277, 22109156) and the China Postdoctoral Science Foundation (2021M703129) for financial support. L. Ding thanks the National Key Research and Development Program of China (2017YFA0206600) and the National Natural Science Foundation of China (51773045, 21772030, 51922032, and 21961160720) for financial support.