1. Introduction
Since the invention of the first electric contact transistor in 1947, the research journey of integrated circuits has been launched. Among them, the invention of metal−oxide−semiconductor field-effect transistors has brought about the rapid development of large-scale integrated circuits. Thanks to the joint progress of device size and chip area, the famous Moore's Law has been obtained, that is, the integration will be doubled every 18 months; when the metal−oxide−semiconductor field-effect transistor (MOSFET) tube size is reduced by x2 times, the speed is increased by x times, and the power consumption is reduced to 1/x2. However, as the device size developed to the nanoscale, quantum effects gradually emerged, and Moore's Law began to fail, and traditional theoretical technologies encountered bottlenecks. Therefore, facing the urgent need for high-performance chips in various emerging application scenarios, it is urgent to find new materials, preparation processes, device structures and their physical mechanisms[1, 2] .
During this exploration process, nanosemiconductors have gradually emerged as the next-generation key technology to breakthrough the field of microelectronics. Among them, various one-dimensional semiconductor materials have become one of the key research areas for the sustainable development of semiconductor technology in the post-Moore era due to their excellent photoelectric properties and potential for building integrated nanodevices[3−8]. In particular, the one-dimensional single-crystal wide bandgap Ⅱ−Ⅵ semiconductors possess excellent optoelectronic properties. Among them, CdS has a direct bandgap width of 2.47 eV. If Sn with different concentrations is doped into the one-dimensional CdS micro-nano structure, it can not only compensate for the shortcomings of the undoped one-dimensional CdS micro-nano structure, such as its single crystal structure, low defect density, and poor insulation, but also broaden the spectral response range, improve conductivity, enhance photoluminescence properties, and introduce new energy levels. Furthermore, its band structure, optical, and electrical properties can be tuned by controlling the doping concentration[9]. In addition, the preparation of Sn-doped CdS with different structures is also simple. These excellent properties all demonstrate its uniqueness and importance. In recent years, numerous scholars have focused on studying various optoelectronic properties of Sn-doped CdS with different micro-nano structures, such as electronic state structure[10], electron−phonon coupling[11, 12], intra-band and inter-band relaxation[13], nonlinear effects[14], Auger recombination process[15], and optical gain characteristics[16]. This is because at the nanoscale, many carrier interaction mechanisms, such as electron−hole plasma, electron−phonon coupling, exciton−phonon scattering, exciton−exciton interaction, and exciton polariton, generate new modes of interaction that affect optical and electrical properties[17−22]. Therefore, a deep understanding of the optical, electrical properties, and luminescence mechanisms of Sn-doped CdS with different micro-nano structures holds significant guiding significance for the further development and research of low-dimensional semiconductor nanomaterials.
Sn-doped CdS exhibits various micro-nano structures, including nanobelts, nanowires, comb-like structures, and superlattices, which give rise to numerous novel semiconductor optical microcavities[23−25]. The interaction between light and matter within these microcavities can alter the original radiative properties and propagation behavior of photons, making them crucial for applications such as low-threshold, high-power semiconductor lasers and photodetectors[26−30]. Additionally, the optical properties, sensitivity, and precision of certain special structures of Sn-doped CdS materials offer new possibilities for anti-counterfeiting applications, such as photonic barcodes and quick response (QR) codes. However, despite the promising performance of Sn-doped CdS in these applications, there are still challenges in regulating its optical properties. Therefore, research on the micro-nano structures of Sn-doped CdS is of significant importance, providing fundamental support for its application in the new generation of integrated circuits and photoelectric integration technologies. This article will present research results on the influence of preparation conditions on the structure of Sn-doped CdS materials, the effects of different structures on photoelectric physical properties, and the application of integrated devices (as shown in Fig. 1). It aims to provide strong support for the application and development of this material and contribute to the sustainable development of semiconductor technology in the post-Moore era.
2. Influence of different conditions on the preparation of Sn-doped CdS
Samples were primarily prepared using the Sn-catalyzed chemical vapor deposition (CVD) method, and the formation of various nanostructure morphologies and the doping content of Sn ions were controlled by adjusting various condition parameters such as growth temperature, reaction time, heating rate, CdS : SnO2 mass ratio, and gas environment. Fig. 2 illustrates various micro-nano structures of Sn-doped CdS obtained by controlling different growth conditions. This paper also summarizes the research on the photophysical properties and applications of these structures.
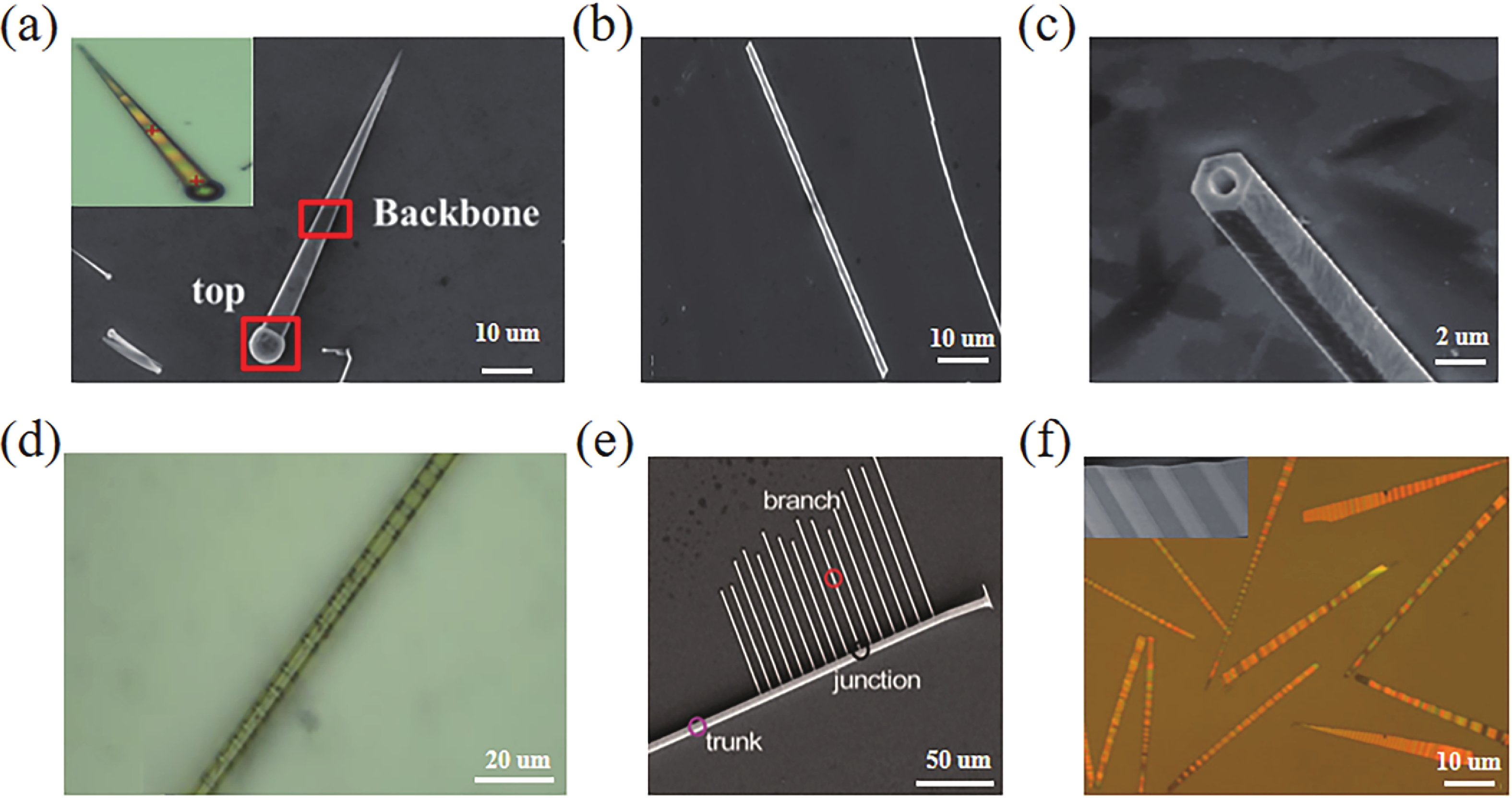
2.1 Temperature and time
Firstly, growth temperature and reaction time are two closely related conditions, and the sample may change due to the length of the reaction time under constant temperature. For the growth time, sufficient growth time contributes to the formation of complete nanomaterials. For example, during the formation of CdS nanotubes, Sn-doped CdS core/shell nanowires were obtained at a reaction time of 15 min at 900 °C, while nanotubes were obtained at a reaction time of 30 min[32]. The growth temperature affects the preparation rate, as a higher growth temperature accelerates the supersaturation formation of Sn−CdS alloy droplets, leading to faster growth rates[35]. Additionally, it also affects the evaporation rate of the material, so different nanostructures can be obtained by modifying other experimental methods based on this point. For instance, Zhang et al.[36] utilized this principle to prepare a flag-shaped CdS nanostructure in 2021. During the growth of CdS/CdS : SnS2 superlattice nanowires, if the temperature is reduced to 900 °C, a series of Sn spheres will appear in the CdS nanowires. However, if the temperature is relatively high, reaching 1050 °C, Sn metal will precipitate as Sn spheres at the ends or sides of the nanowires, unable to form a series of SnS2 layered structures along the axial direction of the nanowires[37]. Furthermore, for the growth of CdS nanotubes, a lower growth temperature is required due to the auxiliary growth of Sn metal wires inside the CdS shell layer. The growth temperature also affects the morphology of the structure. For the preparation of Sn : CdS1−xSex superlattice microwires, regardless of the molar ratio of SnO2 to (CdS + CdSe) in the source powder, the obtained samples exhibited a morphology of thick conical ribbons at temperatures above 800 °C and fine wires at temperatures below 600 °C[38]. Finally, in the last step of the growth of these nanostructures, the temperature needs to be naturally cooled to room temperature to allow the samples to deposit on the Si wafers downstream of the quartz tube.
2.2 Heating-up rate
Heating rate is another crucial factor in the formation of Sn-doped CdS nanostructures, primarily due to its influence on the decomposition rate of SnO₂. In the initial stage of sample preparation, SnO₂ and H2 are overheated and reduced to produce Sn vapor, SnO₂ reacts with H2 through thermal reduction to produce Sn vapor. A faster heating rate enables SnO₂ to be reduced to Sn and completely transformed into Sn vapor within a short time frame, providing a higher Sn vapor concentration for subsequent reactions with CdS vapor[35]. Additionally, the heating rate significantly impacts the nanostructure formation because it controls the rate of oxygen atom release from the decomposition of SnO₂. Zou et al.[39] discovered in 2017 that the heating rate can influence the composition of the finally synthesized CdS nanowires during this catalytic CVD growth process. If the temperature is raised to 1000 °C at a rate of 40 °C/min and maintained for 60 min, red nanowires can be obtained. Conversely, if the heating rate exceeds 80 °C/min, ordinary yellow CdS nanowires are ultimately produced.
The main reason for this phenomenon is illustrated in Fig. 3(b). The oxygen expelled from the growth lines requires even higher temperatures or longer heating times, resulting in the majority of O atoms remaining primarily in the shell region to form CdO domains. On the surface of the CdS nanowires, CdO gradually increases as O is expelled from the wires, akin to the slow accumulation of CdO clusters on the wire surface. Ultimately, with the growth of CdS, they eventually formed a core-shell structure covered by a CdO layer with a CdS inside. This core-shell structure is determined by the interaction of the growth habits of equivalent ion poly-lattices. The heating rate affects this phenomenon because CdS nanowires doped with low concentrations of O (<2%) appear yellow, while those with O concentrations of 5%−20% appear red. CdS nanowires are typically synthesized within the range of 800−850 °C, while the precursor SnO2 powder decomposes at 1000 °C. A rapid heating rate leads to the decomposition of most SnO2, releasing a large amount of oxygen atoms rapidly and expelling them through the tube, whereas a slow heating rate allows oxygen to be gradually released and participate in the reaction process to form a CdO shell. If the heating rate is further increased, the Sn metal core will directly rupture from the CdS nanostructure, yielding a new nanostructure.
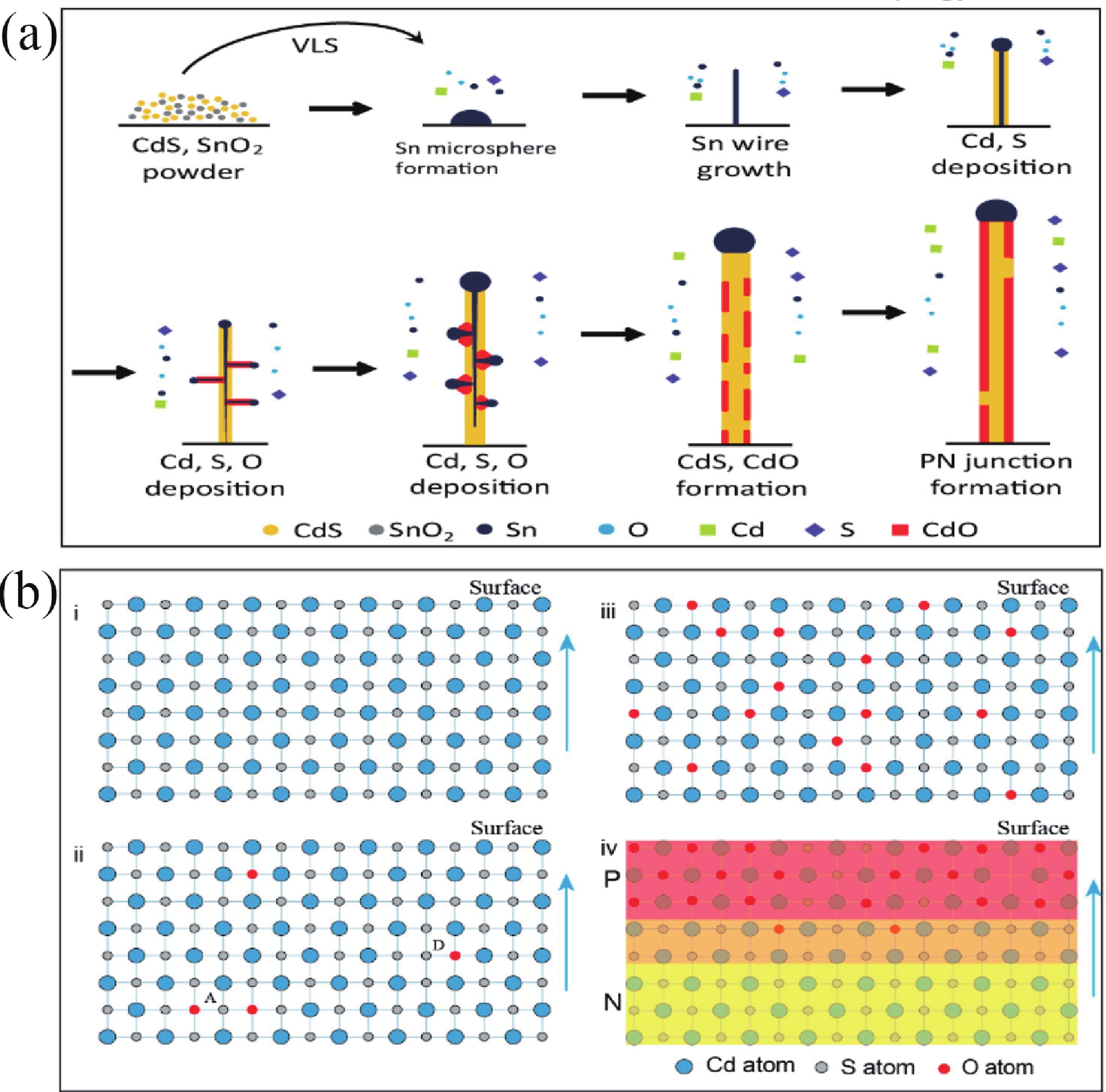
2.3 Stoichiometric ratio
The mass ratio of CdS to SnO2 is primarily responsible for regulating the content of Sn in the doped nanostructure, rather than determining the structure of the sample. This mass ratio can also adjust the wall thickness dimension during the growth process of nanotubes. When the content of SnO2 is too low, a series of pores may appear on the sidewall of the nanotubes due to the excessively thin wall thickness. Regarding the gaseous environment in the reaction, the structure formed is primarily altered by adjusting the hydrogen content. High-purity mixed gas is generally passed through the quartz tube at a fixed flow rate for a period of time before the reaction to purify the environment. During the reaction, the hydrogen in the gaseous environment reacts with SnO2 in the precursor powder (excluding the preparation of CdS/CdO core/shell structures), reducing it to Sn metal. When Sn metal is excessive, it deposits on the surface of the nanowires, promoting the formation of different heterostructures through its catalytic and templating roles[32, 35, 37, 39, 40]. Table 1 presents the experimental condition parameters for the preparation of various samples, including CdS/CdS : SnS2 superlattice nanowires, Sn/CdS core/shell nanowires, CdS nanotubes, and others, using Sn-catalyzed CVD method.
Sample | CdS : SnO2 | Gas environment | Heating rate (°C/min) |
Growth temperature (°C) |
Reaction time (min) |
Superlattice nanowire[37] | 10 : 1 | H2/Ar 10% | 120 | 980 | 20−40 |
CdS/CdO core/shell nanowire[39] | 1 : 1 | Ar | 40 | 1000 | 60 |
Comb-like nanostructure[40] | 16 : 1−10 : 1 | H2/Ar 10%−12% | 70−100 | 1000 | 40−60 |
Nanowire[35] | 10 : 1−0 | H2/Ar 5% | 100 | 1000 | 30 |
Core/shell nanowire[32] | 20 : 1−10 : 1 | H2/Ar 10% | 90 | 900 | 15 |
Nanotube[32] | 20 : 1−10 : 1 | H2/Ar 10% | 90 | 900 | 30 |
3. The photophysical properties of Sn-doped CdS nano-structures
3.1 Nanowire
The Sn-doped CdS nanowire structures are mainly classified into ordinary structures and CdS/CdS : SnS2 superlattice structures. Figs. 4(a) and 4(b) show the Raman scattering spectra of individual Sn-doped CdS nanowires with different dopant concentrations at room temperature. The two strong peaks at 296 and 592 cm−1 are attributed to the 1 LO and 2 LO modes of CdS, respectively[41]. There is a strong coupling between the polarizons along the Z-axis and electrons or excitons. In 1D semiconductor structures, the strength of electron−LO phonon coupling can be significantly enhanced due to phonon confinement in the lateral direction and facile transport of elementary excitations (excitons and photons) in the longitudinal direction. The strength of electron−phonon coupling in semiconductors can be evaluated by the intensity ratio of the overtone mode to the fundamental mode (I2LO/I1LO). The value for Sn−CdS nanowires is 1.67, while it is 1.52 for pure CdS nanowires, indicating a stronger electron−phonon coupling in CdS nanowires doped with Sn. Additionally, impurity vibration modes such as Eg, A1g, A2u(TO), and A2u(LO) associated with doped SnS2 can also be observed in the Raman scattering spectra[42]. Among them, A2u(TO) and A2u(LO) are infrared-active modes of SnS2, indicating significant relaxation of transition rules with the assistance of electrons[43]. Furthermore, doping with Sn leads to increased crystal deformation and surrounding structural fluctuations in CdS nanowires, partially accounting for the weak activity of infrared modes[44]. Additionally, it can be observed in the Raman spectra that the intensity ratio of CdS modes to SnO2 modes is inversely proportional to the ratio of SnO2 to CdS in the source powder. Therefore, the relative doping concentration of Sn can be qualitatively determined based on the Raman scattering spectra.
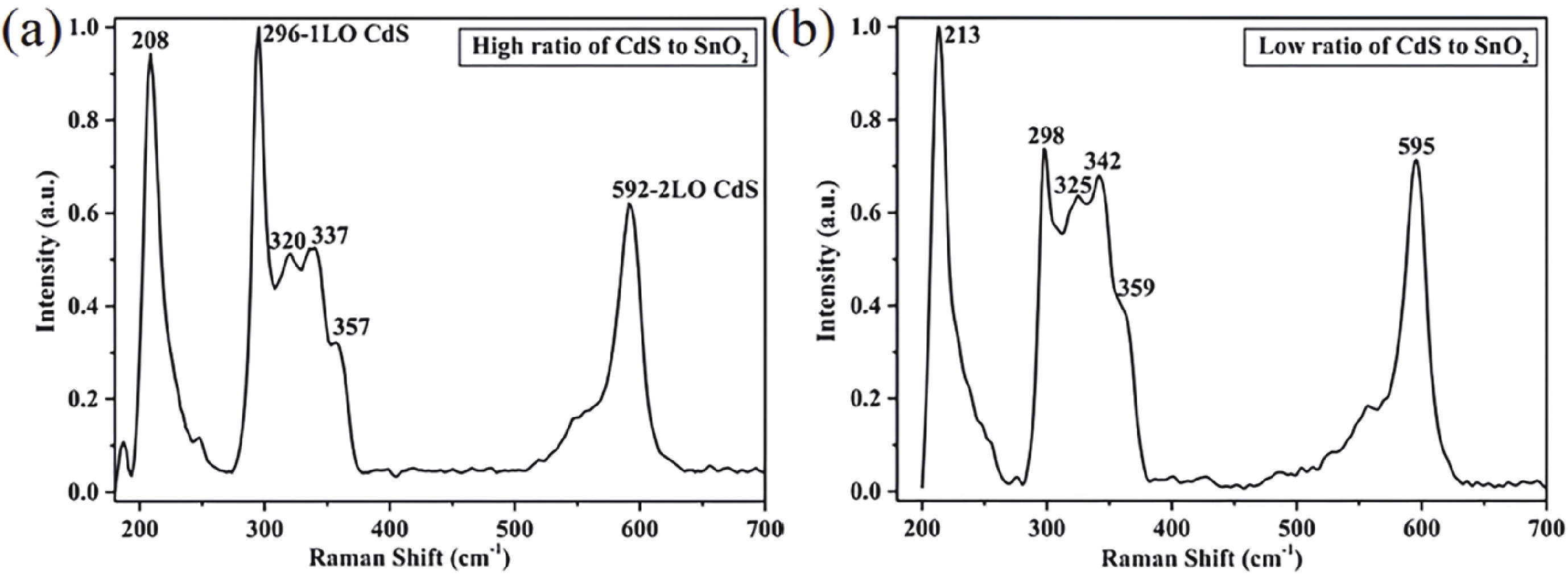
By analyzing the intensity ratio between the near-bandgap emission and the trapped state emission in the PL spectra of Sn-doped CdS nanowires, the relationship between Sn doping effects and electron−phonon coupling can be explored. As shown in Fig. 5(a), the emission at 509 nm originates from the near-bandgap emission, while the broad emission band at lower energy originates from the trapped state state emission. With the increase in excitation power, the intensities of all emission bands increase rapidly, and the increase rate of the near-bandgap emission band is only slightly faster than that of the trapped state state emission. This phenomenon differs from the traditional deep trap states, where the intensity of near-bandgap emission increases significantly with the increase in excitation power. The multi-phonon spectrum of the trapped state state indicates its nonlinear electron−phonon coupling. The number of multi-phonon scattering processes (n) is proportional to the polaron coupling coefficient α, i.e., the maximum frequency shift nωLO is proportional to the deformation potential energy (0.5 αhωLO)[46]. Therefore, the current PL spectra suggest that there exists a large deformation potential energy and strong electron−phonon coupling in Sn–CdS nanowires.
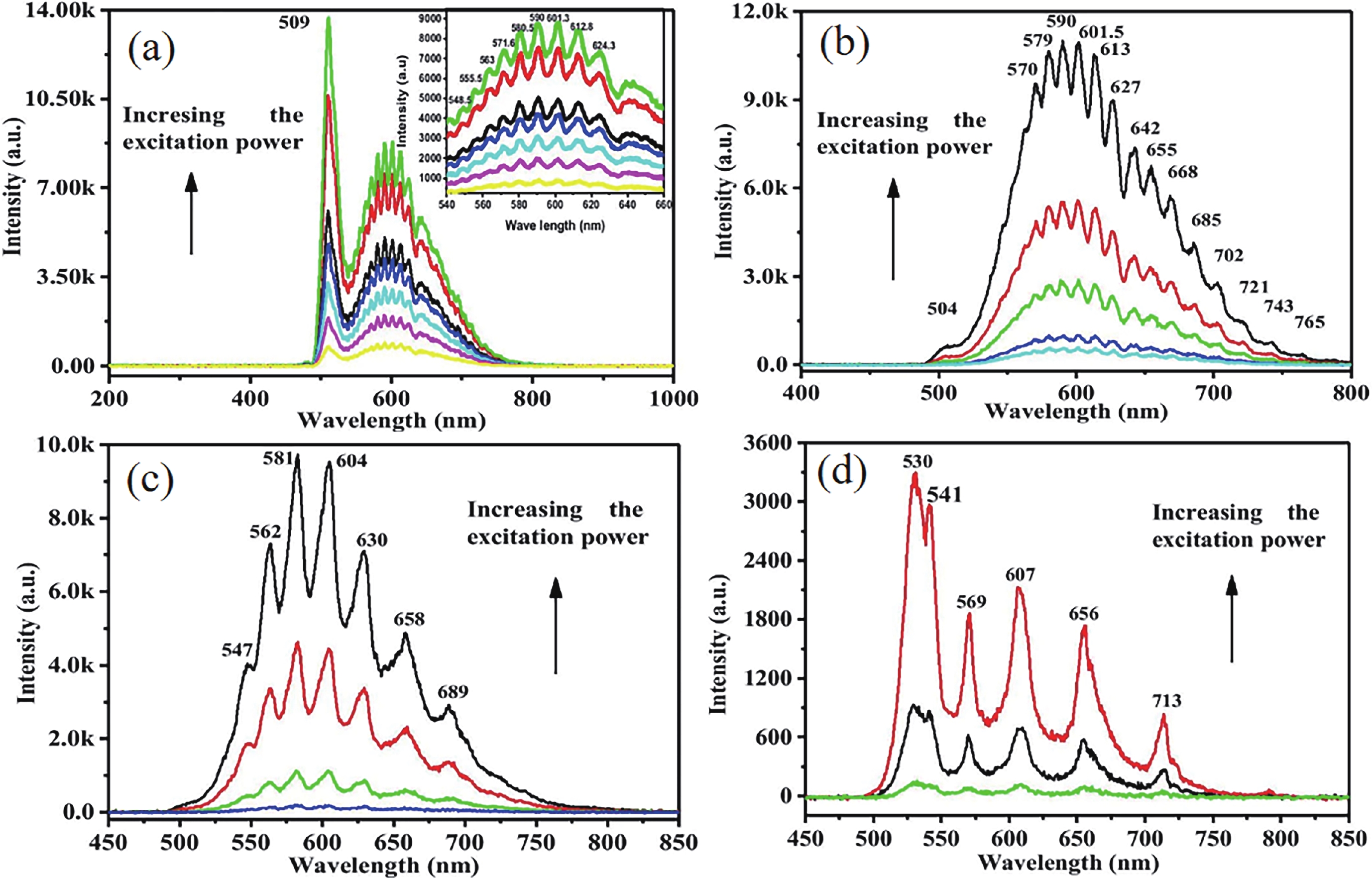
As the concentration of Sn(Ⅳ) doping in CdS nanowires gradually increases, the overall intensity of the PL spectra decreases, but the near-bandgap emission decreases much more relative to the impurity emission, indicating that electron−phonon coupling strengthens with the increase in doping concentration. Figs. 5(a) and 5(b) show the PL spectra obtained after gradually increasing the Sn doping concentration. From Figs. 5(a) and 5(b), the energy span between adjacent impurity emission bands increases to nearly the LO phonon energy (296 cm−1), demonstrating that more Sn ions are doped into the nanowires to produce stronger electron−phonon coupling. Furthermore, unlike Fig. 5(a), in Fig. 5(b), as the excitation power increases, the increase rate of the trapped state emission intensity is faster than that of the near-bandgap emission. This phenomenon indicates that the scattering effect of impurities and phonons on the trapped state emission is relatively stronger. In Fig. 5(c), the span increases slightly to nearly the 2LO phonon energy. The generation of such bound pairs resembling biexcitons in electron−LO phonon coupling may be due to the formation of Sn−Sn pairs under high doping conditions. This biexciton (electron−2LO phonon) state can emit coherent light, typically occurring at defect centers with long lifetimes, while the 1LO-assisted emission band becomes indistinct after long-distance transmission and short-time relaxation. Therefore, 2LO-assisted emission may dominate in nanowires with higher doping concentrations. In Fig. 5(d) with a higher Sn doping concentration, the energy span approaches the 4LO phonon energy (1184 cm−1). This frequency-doubling effect indicates the existence of higher-order electron−phonon coupling in Sn−CdS nanowires[45].
Fig. 6 shows the waveguide images of nanowires under laser excitation (488 nm). Figs. 6(a) and 6(b) depict a single cadmium sulfide nanowire and multiple interconnected nanowire structures, respectively. The bright spots indicated by the red arrows in the figures represent the luminescent positions under laser excitation. The emitted light can effectively propagate along the nanowires and emit at their ends (white arrows). During the transmission process, there is varying degrees of energy loss, particularly coupling loss at the junction areas in interconnected structures, scattering loss due to defects, and radiation loss caused by bends on the nanowires. Nevertheless, even with these losses, the light can effectively propagate for approximately 110 μm and then emit at the end of another interconnected nanowire. This is because when the laser irradiates the nanowire, it generates a large number of excitons that propagate along the c-axis, forming a mixed state of excitons and photons[35]. This coupled state has a higher binding energy than free excitons[47], enabling higher stability at room temperature and longer propagation distances within the nanowire. Fig. 6(c) presents the PL spectra at the excitation end (a) and emission end (b) of a nanowire structure without bends, allowing for the determination of losses and red shifts during transmission. The long-distance and high-efficiency optical waveguide properties of Sn-doped CdS nanowire structures are mainly attributed to strong defect state emission, providing a foundation for the study of nanophotonic devices[48].
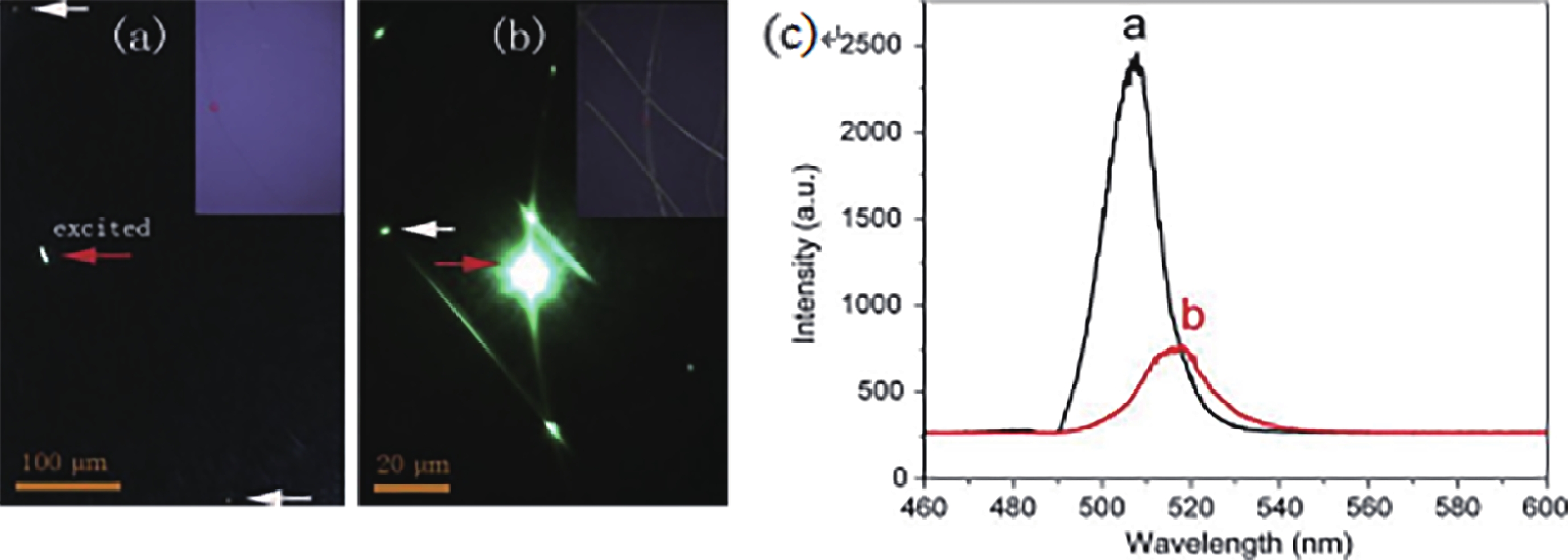
Guo et al.[49] conducted a study on the optical waveguide losses in nanowires in 2020. Figs. 7(a) and 7(b) show the distance-dependent near-band-edge emission (NBE) and deep-level emission (DLE) intensities under different laser power densities. These data can be fitted using an exponential decay function I = I0e−αx, where I represents the output emission intensity collected at the end of the nanowire, I0 is the emission intensity at the excitation point, α is the decay coefficient, and x is the propagation length. The decay coefficients for NBE and DLE can be adjusted by varying the excitation power density. As the power density increases from 27.6 to 660 kW/cm2, the fitting coefficient for NBE decreases from 0.097 to 0.061 μm−1, while the decay coefficient for DLE decreases from 0.098 to 0.067 μm−1, which is lower than that for NBE. Therefore, the transmission efficiency of Sn-doped CdS nanowires is an adjustable parameter.
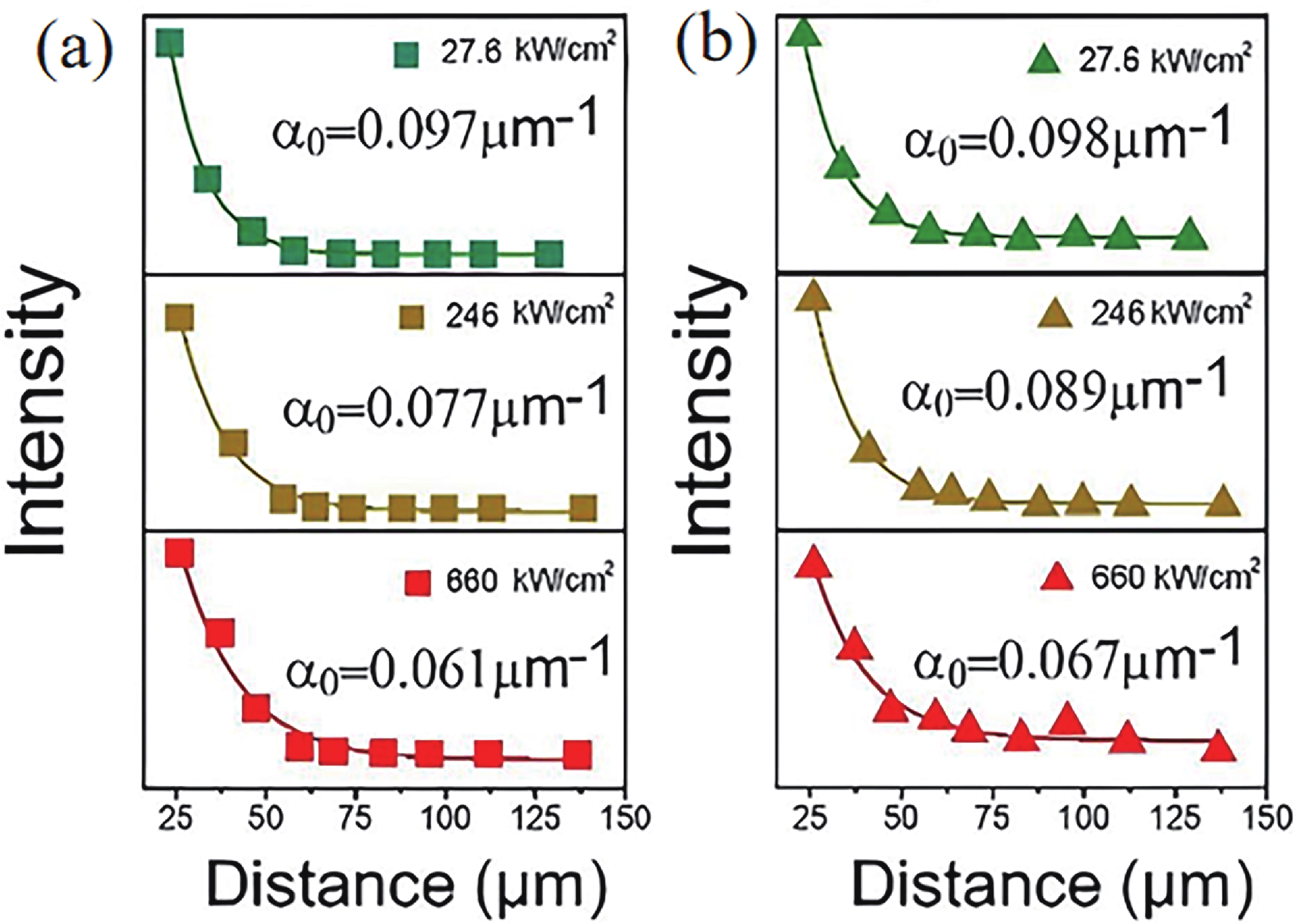
When excited by a laser, Sn-doped CdS nanowires exhibit lasing phenomena, and the lasing threshold is influenced by different gaseous environments. In atmospheric conditions, the lasing threshold is approximately 10.5 MW/cm2, while in argon, nitrogen, and helium environments, the thresholds are 9.82, 8.25, and 7.22 MW/cm2, respectively. It can be observed that the gaseous environment has a certain impact on reducing the lasing threshold of the nanowires. As the mass of gas molecules decreases, the lasing threshold gradually decreases. Especially in a helium environment, the lasing threshold decreases from 10.5 to 7.22 MW/cm2. This is because gas molecules can be ionized under strong excitation, generating high-temperature plasma. Therefore, the changes in the lasing threshold of nanowires in different gaseous environments are influenced by the transient polarization of gas molecules. Under strong excitation power, gas molecules are ionized to form plasma. The gas plasma surrounding the nanowires becomes polarized due to the influence of electrons on the inner surface of the nanowires, adsorbing onto the nanowire surface and resulting in a slow decay of hot electrons in the nanowires, achieving population inversion. The smaller the mass of gas molecules, the more polarized molecules adsorb onto the nanowire surface, leading to the easier generation of more population inversion and, thus, a more prone lasing phenomenon.
Under low-temperature conditions, Sn-doped CdS nanowires exhibit novel optical properties, which were investigated by Zhang et al.[9] in 2014 through photoluminescence (PL) spectroscopy. As shown in Fig. 8(a), three spectral emission regions of the nanowires at 7 K are observed: near-band-edge (NBE) emission at 2.55 eV, arising from exciton emission; deep-level emission (DLE) at 2.1 eV, attributed to several defects such as carrier recombination at Cd crystal sites[50]; and the region between 2.45 and 2.2 eV, where DAP and a transition from a free state to a bound state (e, A) occur along with their phonon replicas. These peaks do not occur in pure CdS, suggesting that they may be induced by Sn doping. Fig. 8(b) presents further observations of the NBE transition, as well as DAP and (e, A) transitions, at 4 K, which are strongly accompanied by phonon replicas with distinct spacings. Spectral measurements at different temperatures provide further insights into the properties of the nanowires. As shown in Fig. 8(c), with increasing temperature, the NBE exhibits a red shift to lower energies due to the "Varshni shift"[51]; while the double structure of DAP and (e, A) shifts towards higher energies by approximately 7 meV. This blue shift may originate from the thermal occupation of higher donor energy levels, attributable to both intrinsic and Sn-induced donor states. The ionization of donor−acceptor pairs has a corresponding activation energy of 18 meV, resulting in a Boltzmann factor N of approximately 3 × 10−3 at 35 K. However, thermal ionization is much less significant than the thermal occupation of higher donor states, as ΔED is approximately 7 meV and N is approximately 0.1. Therefore, a significant number of electrons occupy these higher donor states. Fig. 8(d) summarizes the properties of these energy levels, including near-band-edge emission composed of exciton emission, electron−hole coupling pairs, and the double structure of donor−acceptor pairs and deep-level emission.
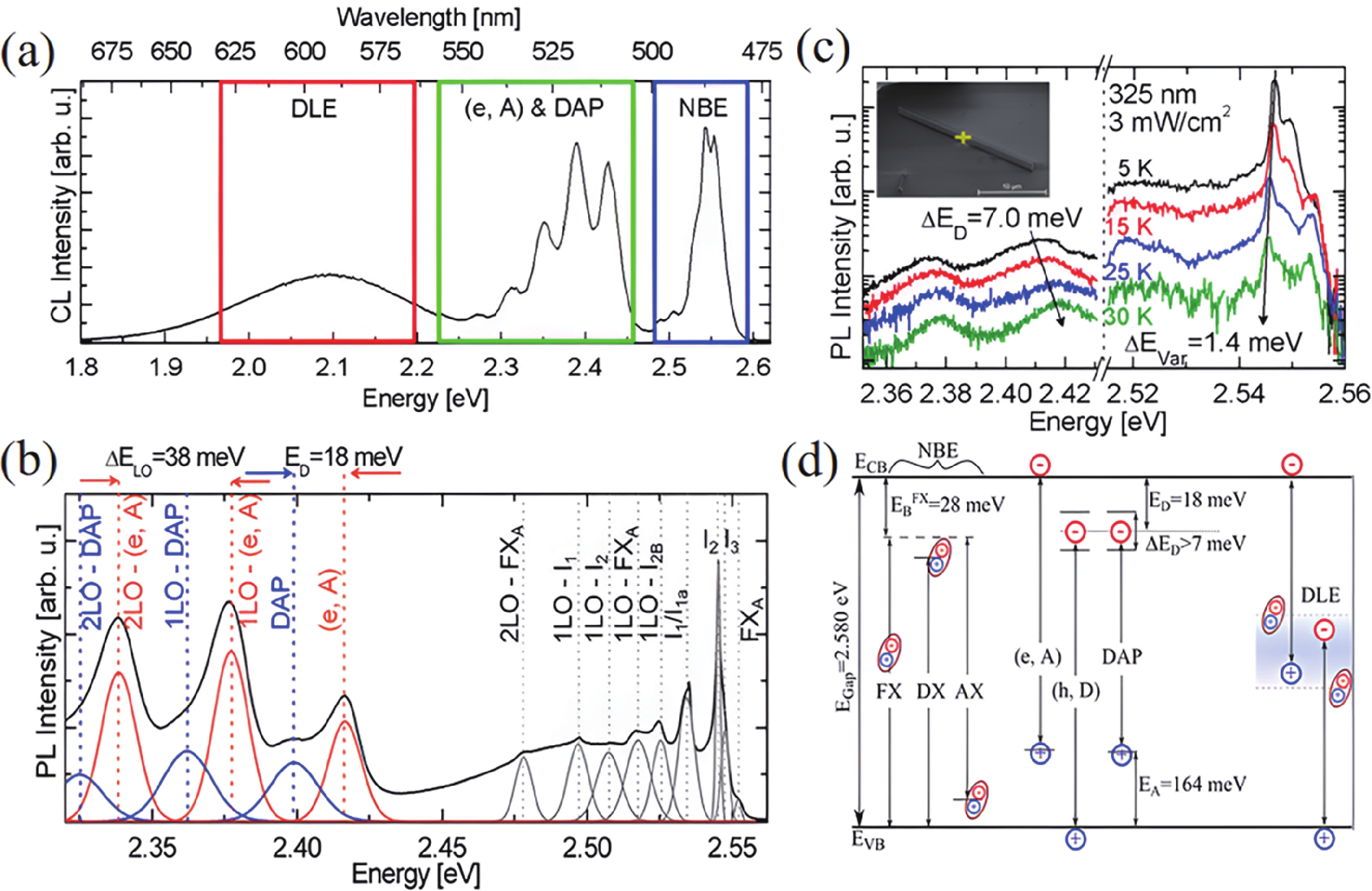
Zou et al.[52] also conducted relevant research in 2019. Figs. 9(a) and 9(b) respectively show the real images of Sn-doped CdS nanowires at different temperatures and their corresponding PL spectra. As the temperature decreases, the luminescent spots shown in Fig. 9(a) become brighter, indicating a stronger luminescent effect in the nanowires. When the temperature drops to 80 K, three new luminescent spots appear, corresponding to the new emission peak at 525 nm in the PL spectrum, representing an enhanced longitudinal light propagation effect. According to the research by Dai et al.[37], these new luminescent spots and their corresponding peaks are related to the emission of SnS2. The structure of the SnS2 layer can produce a local response reflection effect on longitudinal luminescence, acting as an Fabry−Perot (F−P) cavity for longitudinal luminescence. However, the oscillation mode of the F−P cavity is not as significant as the WG oscillation. Sn2+ and Sn3+ ions may disperse in the nanowires and generate bound carriers, affecting gap luminescence. As the temperature decreases, the intensity of band-edge emission and gap emission increases, and a set of WGM resonance peaks are observed in the long-wavelength region, as shown in Fig. 9(b).
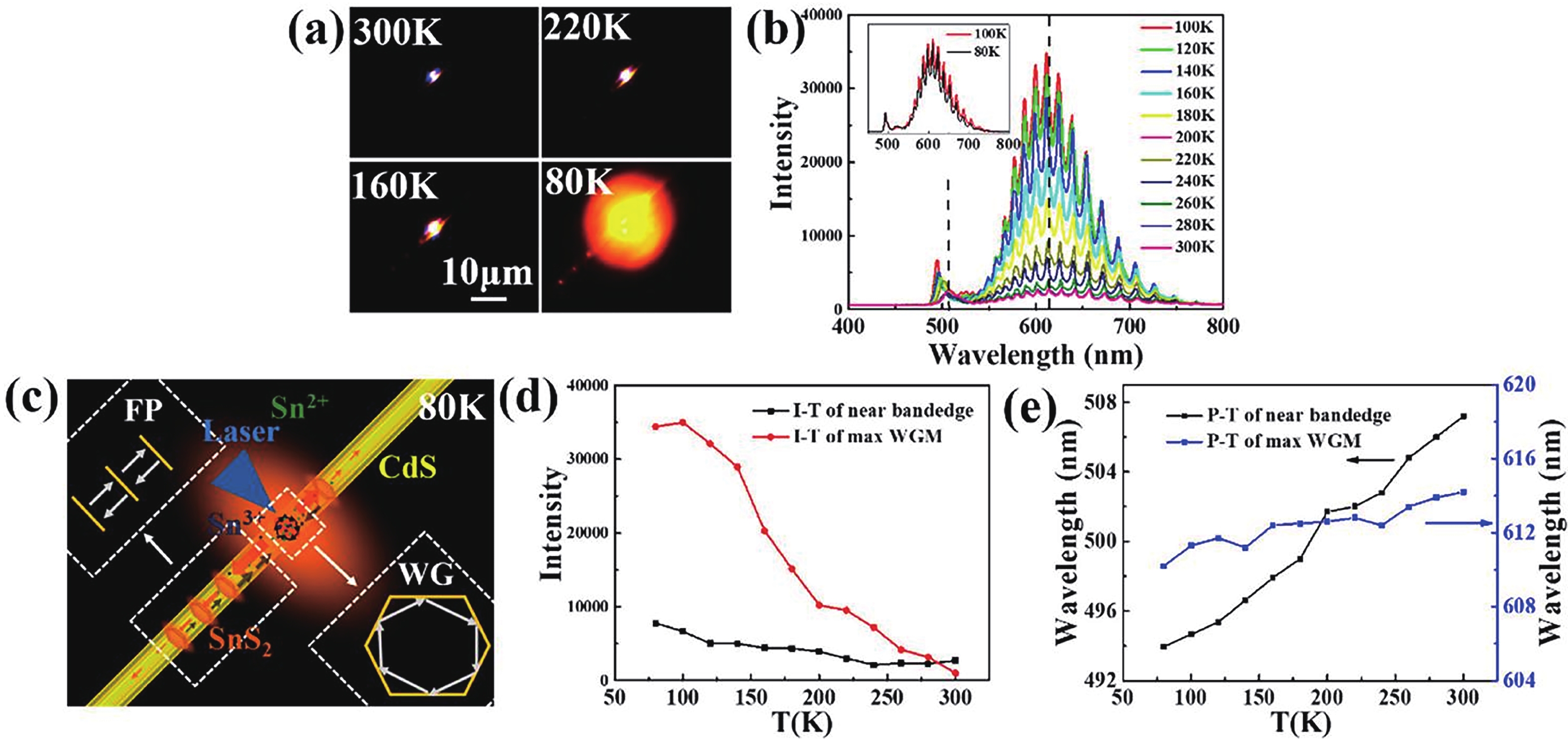
As the temperature decreases, the luminescence intensity between the energy bands gradually increases from below the band-edge luminescence intensity to approximately seven times the band-edge luminescence intensity at 80 K. This may be due to the enhancement of the microcavity effect in Sn-doped CdS nanowires at low temperatures, where the combined effects of local F−P and waveguide (WG) cavity resonances contribute to light enhancement. Additionally, Figs. 9(d) and 9(e) present a detailed analysis of Fig. 9(b), showing that the intensity of the near-band-edge emission remains relatively unchanged, with a linear red shift in its peak position as the temperature increases. The 525 nm peak of SnS2 serves as a dividing point, with light above this wavelength being absorbed and emitted, while light below it is reflected, resulting in the enhancement of the F−P cavity and the promotion of interband emission enhancement in conjunction with the WG cavity. Furthermore, the decrease in temperature weakens scattering relaxation, leading to a significant increase in the intensity of the maximum WGM peak as the temperature decreases, with minimal changes in the wavelength position. Additionally, when the temperature reaches 100 K, the luminescence of the bound states induced by Sn doping nearly saturates, resulting in a slow change in the intensity of interband emission. The red shift near the band edge at different temperatures represents the behavior of free exciton−phonon scattering. The red shift of the near-band-edge emission at different temperatures represents the behavior of free exciton−phonon scattering, where the enhancement of electron−phonon interactions and lattice expansion with increasing temperature lead to shifts in the conduction and valence bands[53]. Compared to the near-band-edge emission, the red shift of the interband emission is less pronounced because the state energies and microcavity modes associated with the luminescence peaks induced by Sn doping do not undergo significant changes with temperature variations.
The periodic small bright spots observed in Fig. 9(a) at 80 K represent a distinct structure from a pure nanowires sample, namely a superlattice nanowires structure. This structure features uniformly spaced SnS2 layered structures along its axial direction, forming a series of tandem F−P cavities that modulate the luminescence of the nanowires while generating a series of waveguide behaviors at the nodes. In 2010, Dai et al.[37] first synthesized CdS/CdS : SnS2 superlattice nanowires using Sn-catalyzed CVD growth methods. These nanowires exhibit high crystalline quality, with SnS2 layered structures appearing at equal intervals along the growth direction of the CdS nanowires, as shown in Fig. 10.
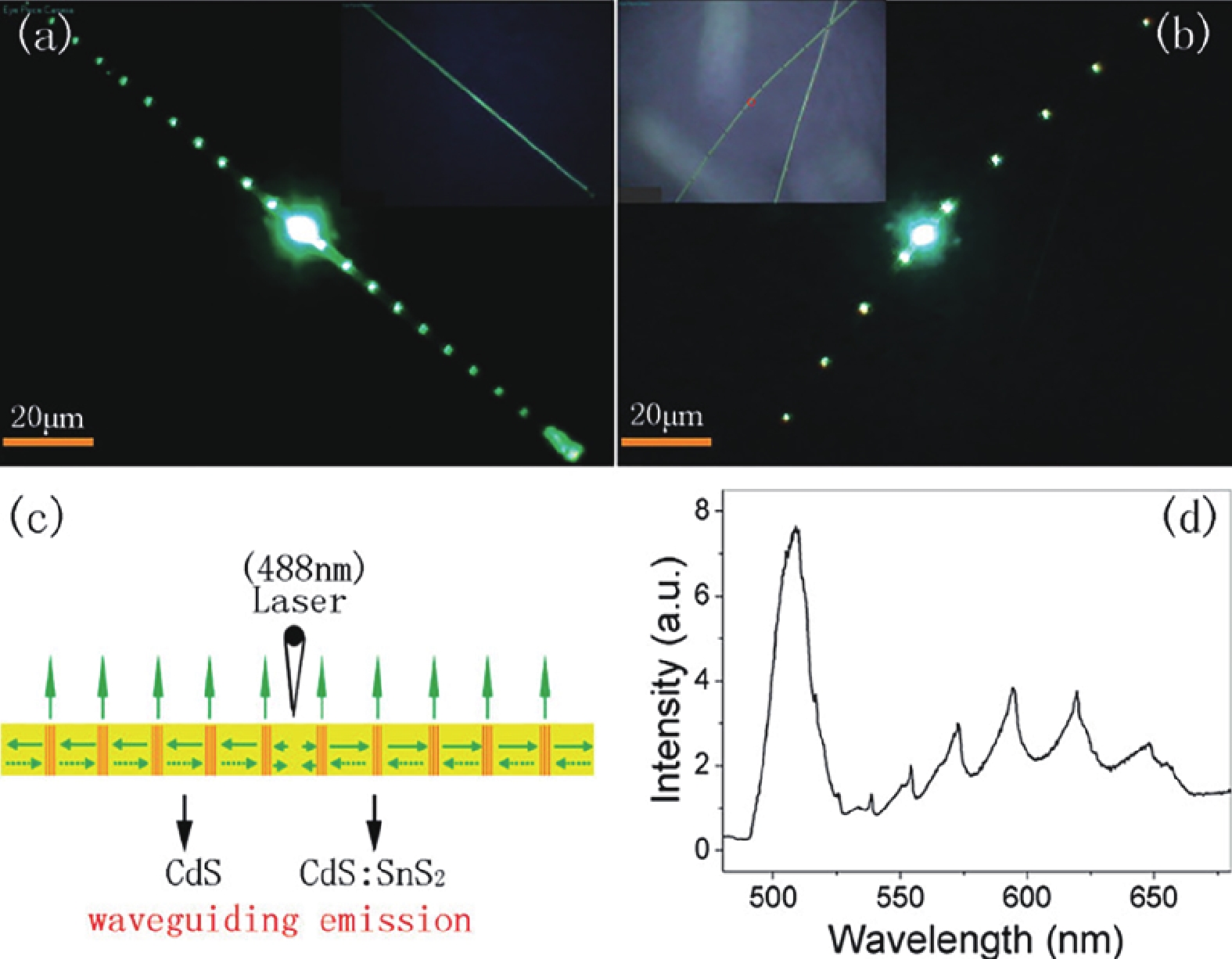
The CdS segments in this novel superlattice nanowire can form numerous periodic optical microcavities that confine and transmit photons, while the SnS2 with a higher refractive index within the short CdS : SnS2 segments serves as both a reflecting end-face and an emission center. The periodic bright emissions arise from the interference of coherently scattered light waves at the microcavity end-faces and the emission from SnS2. Figs. 10(c) and 10(d) depict the emission curve and corresponding PL spectrum of the superlattice nanowire, respectively, exhibiting a strong band-edge emission at 509 nm and a multi-peak range from 525−650 nm. Due to the serial effect of axial F−P cavities, the behavior of excitons and photons can be significantly modulated. In its luminescence spectrum, a series of periodic mode peaks appear across a broad wavelength range, a phenomenon resulting from the exciton confinement in the superlattice nanowire. This structure provides a platform for studying exciton−photon coupling and the microscopic interactions between photons and quasiparticles, and it can also be used to investigate the optical nonlinear effects of tandem microcavities in nanowires.
If CdS1−xSex/Sn : CdS1−xSex superlattice nanowires are prepared by incorporating different amounts of Se elements[38], their PL spectra can exhibit a luminescence intensity approximately 10 times higher than that of ordinary CdS nanowires. Moreover, varying the ratio of Se and S can shift the main band emission spectrum of the nanowires under different deposition temperatures, as shown in Fig. 11(e), where the PL emission peak shifts from 513 to 596 nm. Additionally, multiple small peaks arise at the low-energy side of each main band, resulting from the combined contributions of coupled optical microcavity modes[54] and local excitons within the 1D photonic crystal wires. Figs. 11(a)−11(d) demonstrate the periodic color changes ranging from green to red, which also depend on the ratio of Se and S, further corroborating the multimodal emission and color-tuning optical properties of this structure.
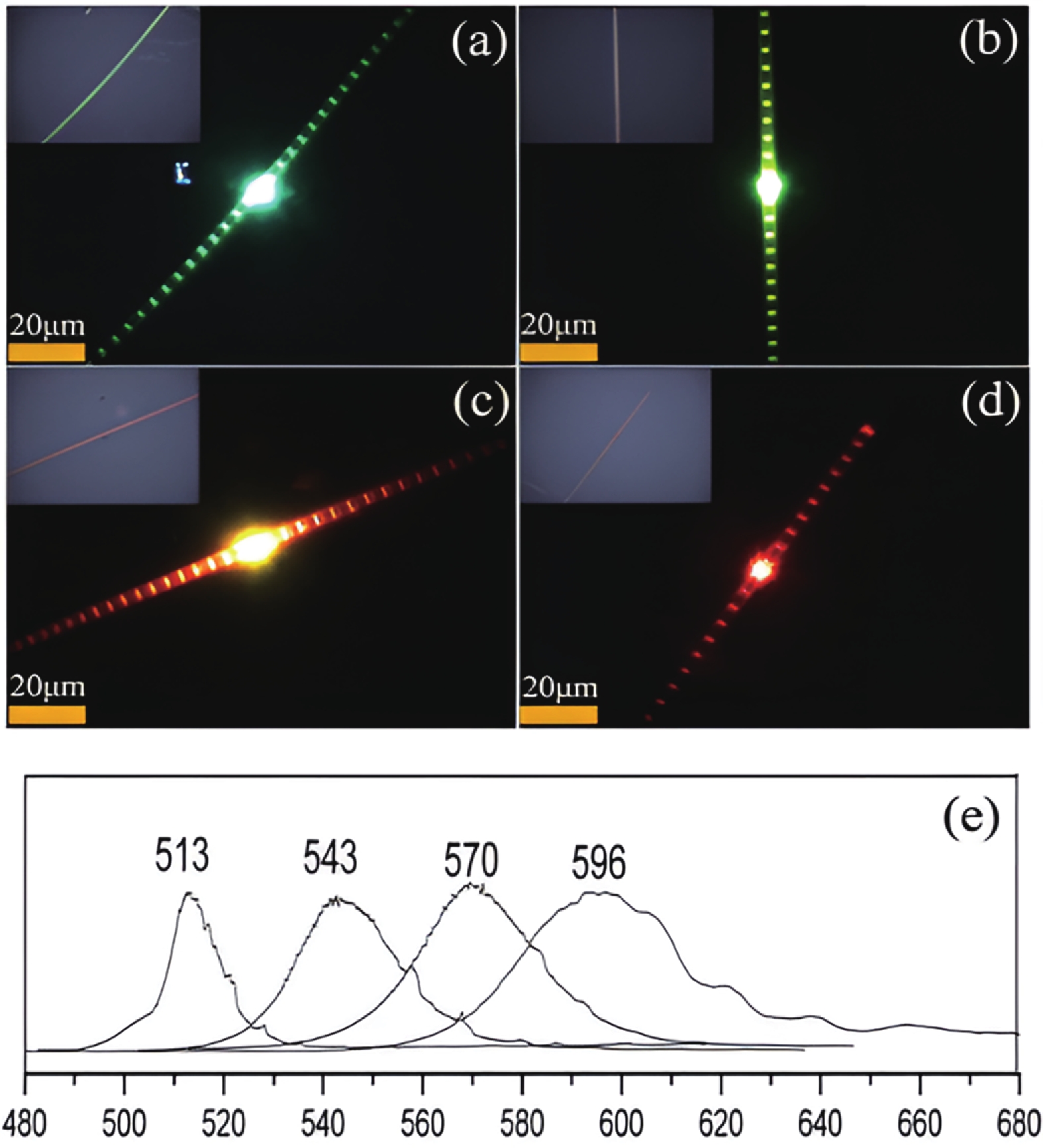
Figs. 12(a) and 12(c) display the photoluminescence images of pure CdS nanowires and CdS/CdS : SnS2 superlattice nanowires, respectively. It can be observed that the pure CdS nanowires serve as highly efficient waveguide microcavities, enabling the transmission of excited photons to their termini and generating luminescence. On the other hand, the CdS/CdS : SnS2 superlattice nanowires exhibit specific periodic emission characteristics. The dark sections correspond to CdS/SnS2 regions, while the bright sections represent CdS regions. This indicates that due to the different refractive indices of SnS2 and CdS, photons propagate periodically along the axial direction of the nanowires, further validating the superlattice structure of the nanowires. When the sample is illuminated by a laser, a significant amount of photons are excited and propagate longitudinally along the nanowires to the interface between CdS and SnS2. Some photons are reflected, while others pass through and continue to propagate. The reflected photons are reflected back to the other interface, where some are again reflected, and some pass through, creating oscillations in this small cavity. Figs. 12(b) and 12(d) show the PL spectra of the two structures, respectively. The intense PL peak at approximately 508 nm in Fig. 12(b) originates from the intrinsic emission at the CdS band edge. Fig. 12(d) exhibits a strong CdS band-edge peak at approximately 508 nm and several weaker SnS2 peaks ranging from 550 to 625 nm. These multiple peaks may be attributed to the SnS2 sections that form whispering gallery mode cavities. The CdS sections form optical waveguide microcavities, facilitating the axial transport and radial confinement of photons. Although the short CdS : SnS2 segments may not simultaneously serve as reflecting end-faces and emission centers due to their larger refractive index, they can still be considered as favorable longitudinal photon propagation segments within the coupled optical microcavities[54].
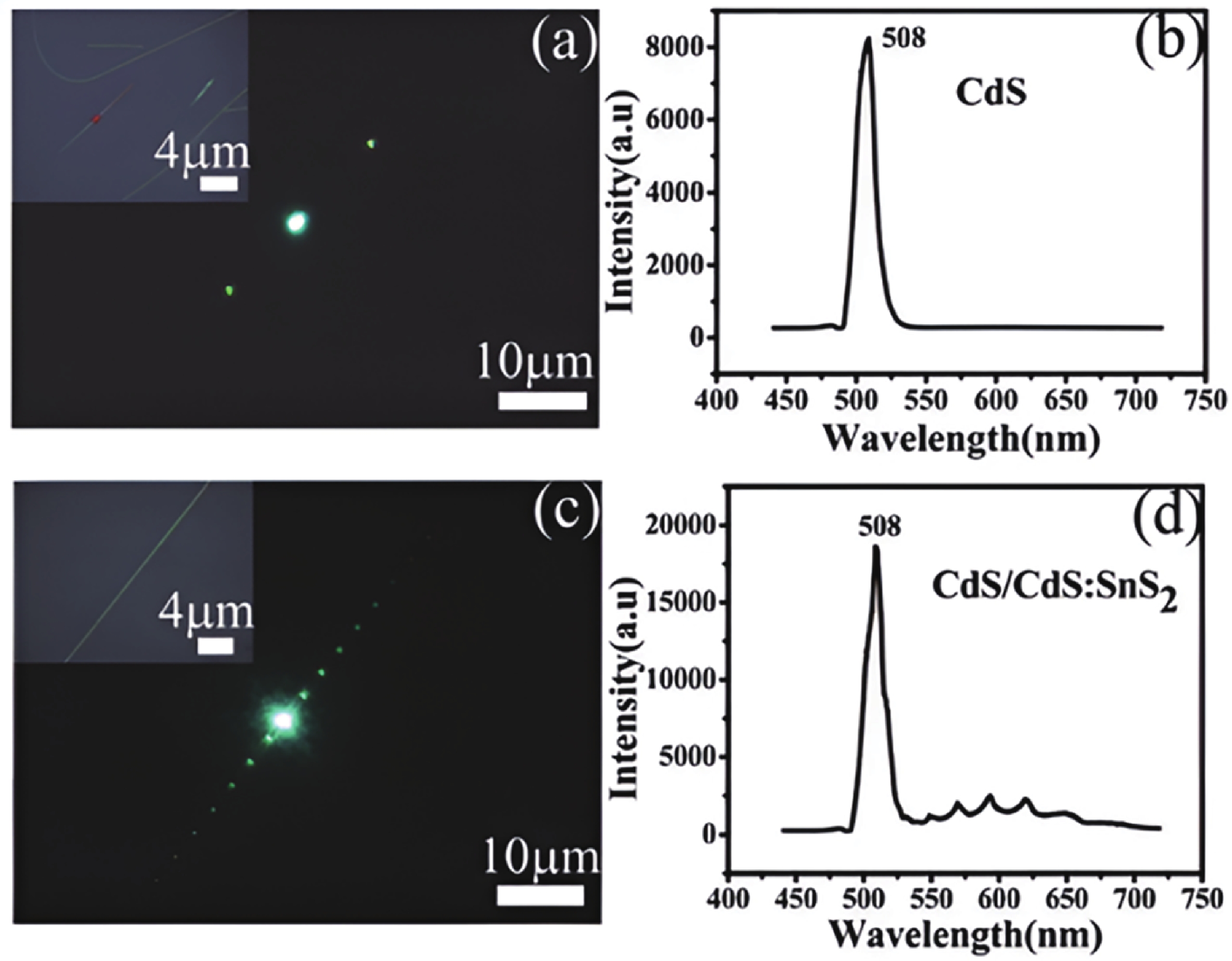
3.2 Superlattice micron cone structure
The superlattice microcone structure of Sn-doped CdS exhibits numerous similarities in photophysical properties to the aforementioned superlattice microwires, primarily due to their identical superlattice structure. However, a significant difference lies in the significant size variation observed in the microcone structure, in which Tian et al.[31] have conducted a series of studies on the novel properties stemming from this factor. Fig. 13(a) presents spectra obtained using different wavelength lasers as excitation sources, revealing multiple oscillatory modulations. The broad emission peaks in the spectra may be associated with defect emissions caused by excessive Sn doping. Additionally, the multimodal emissions excited by three different wavelength lasers exhibit identical spectral shapes due to microcavity emissions. Fig. 13(b) shows excitation power-dependent PL spectra at another position of the sample. The intensity of the multi-peak emissions exhibits a linear growth trend and saturates at a certain power level. When the excitation power reaches a high level, a significant accumulation of carriers occurs in the defect states, hindering further electron capture and energy transfer processes. Notably, as the excitation power increases, the wavelength remains almost unchanged, without significant shifts. This phenomenon aligns with the behavior of traditional optical microcavities, as the influence of excitation power on the microcavity energy is relatively weak. Furthermore, whispering gallery mode microcavities along the diameter direction are formed within the CdS/CdS : SnS2 superlattice microcones. Fig. 13(c) illustrates the emission spectra at ten different positions (with cross-sectional radii ranging from 1.21 to 2.86 μm) within the microcone. The final emission peak wavelengths at the selected positions fall within the range of 560−850 nm, but the spectral shapes vary. A larger cross-sectional radius results in a higher number of resonant modes, with the number of resonant peaks increasing from 3 to 17. Moreover, it can be observed from Figs. 13(a) and 13(b) that the spectral shapes vary at different positions, indicating that the CdS/CdS : SnS2 superlattice microcone can achieve wavelength tuning.
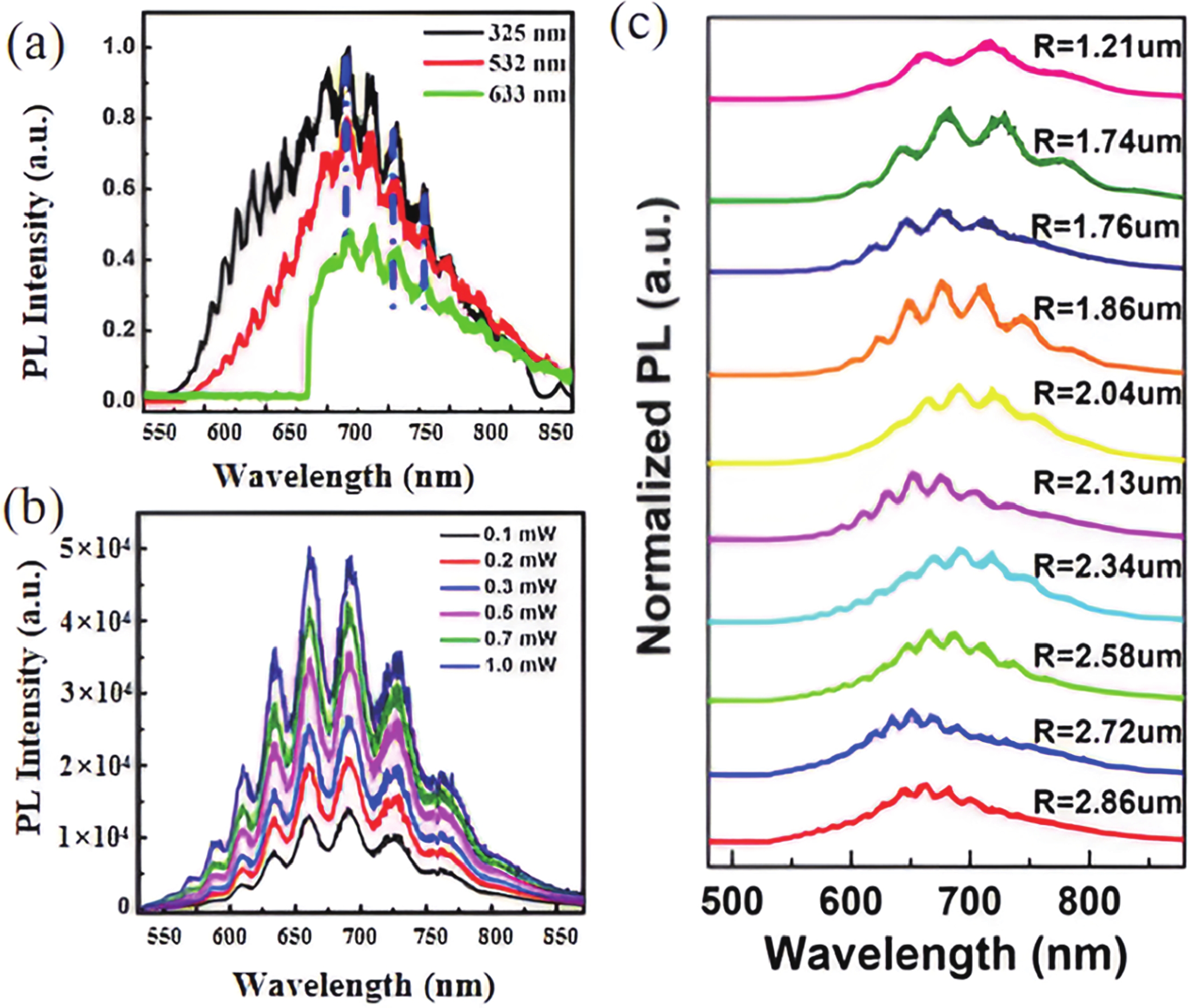
In addition, the spectral properties of free exciton A (FXA) were also studied in the same year. By discussing the influence of slit angle on the sample surface on the polarization-dependent spectral results, the influence of structural anisotropy on FXA and FXB emissions was demonstrated. As shown in Figs. 14(a) and 14(b), the ARPL spectra of the micrometer cone samples are presented when they are perpendicular (TM polarization) and parallel (TE polarization) to the incident slit of the monochromator, respectively. Under TM polarization, both FXB and FXA exhibit distinct emissions. Under TE polarization conditions, FXB emission is evident. Furthermore, compared to pure CdS nanostructures, the FXA intensity is stronger in Sn-doped CdS nanostructures. At 54 mW, the movements of the conduction and valence bands are not significant, resulting in a reasonable quantitative contraction of the radius dependence of the ARPL spectra. Moreover, as the cross-sectional radius decreases, FXA exhibits a blue shift (from 521.6 to 516 nm), while FXB emission remains near 506 nm, as shown in Fig. 14(c). This influence of cross-sectional radius on FXA emission is attributed to the quantum confinement effect induced by size depletion. When the cross-sectional radius is small, the energy difference between FXA and FXB is numerically much smaller than the energy of longitudinal optical phonons in CdS (38 meV), which prevents FXB from relaxing to a lower energy state by transferring energy to longitudinal optical phonons. When the cross-sectional radius is large, the energy difference between FXA and FXB is large, allowing FXB to couple with longitudinal optical phonons and relax to a lower energy state. This is also the reason for the different behaviors of FXA and FXB emission peaks with changes in cross-sectional radius[56]. This finding demonstrates that the emission of FXA can be controlled by manipulating the cross-sectional radius.
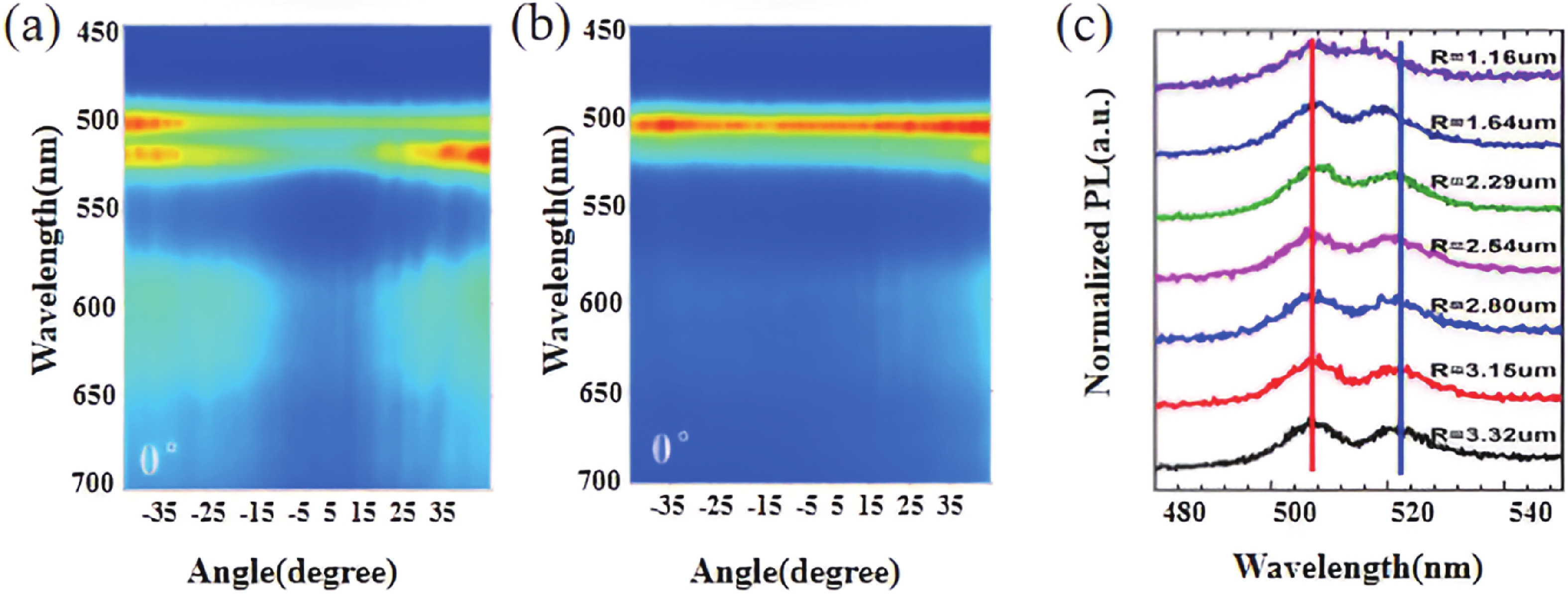
Tian et al. further investigated and discovered a certain relationship between the cross-sectional radius of CdS/CdS : SnS2 superlattice micrometer cone and polarized emission[57]. Fig. 15(a) shows the circularly polarized PL spectra with radii varying from 1.15 to 3.39 μm. Based on the obtained polarized PL spectra, the degree of polarization r was calculated to describe the polarization characteristics at different radii[58]. As shown in Fig. 15(b), the polarization of the micron cone increases from 24.39% to 61.52% with the decrease of the cross-section radius due to the decrease of the luminous lifetime. Typically, the quantum size effect and optical confinement effect are the two main mechanisms used to explain the underlying causes of polarization enhancement. However, the quantum size effect that affects the degree of polarization also influences the emission energy. As shown in Fig. 15(c), the emission peak blueshifts from 504.23 to 503.31 nm. Traditional optical methods provide limited information on different excitons. Considering the shift in emission peaks, it is a challenging problem to explore the reasons for polarized emission in the context of spectral mixing of different excitons.
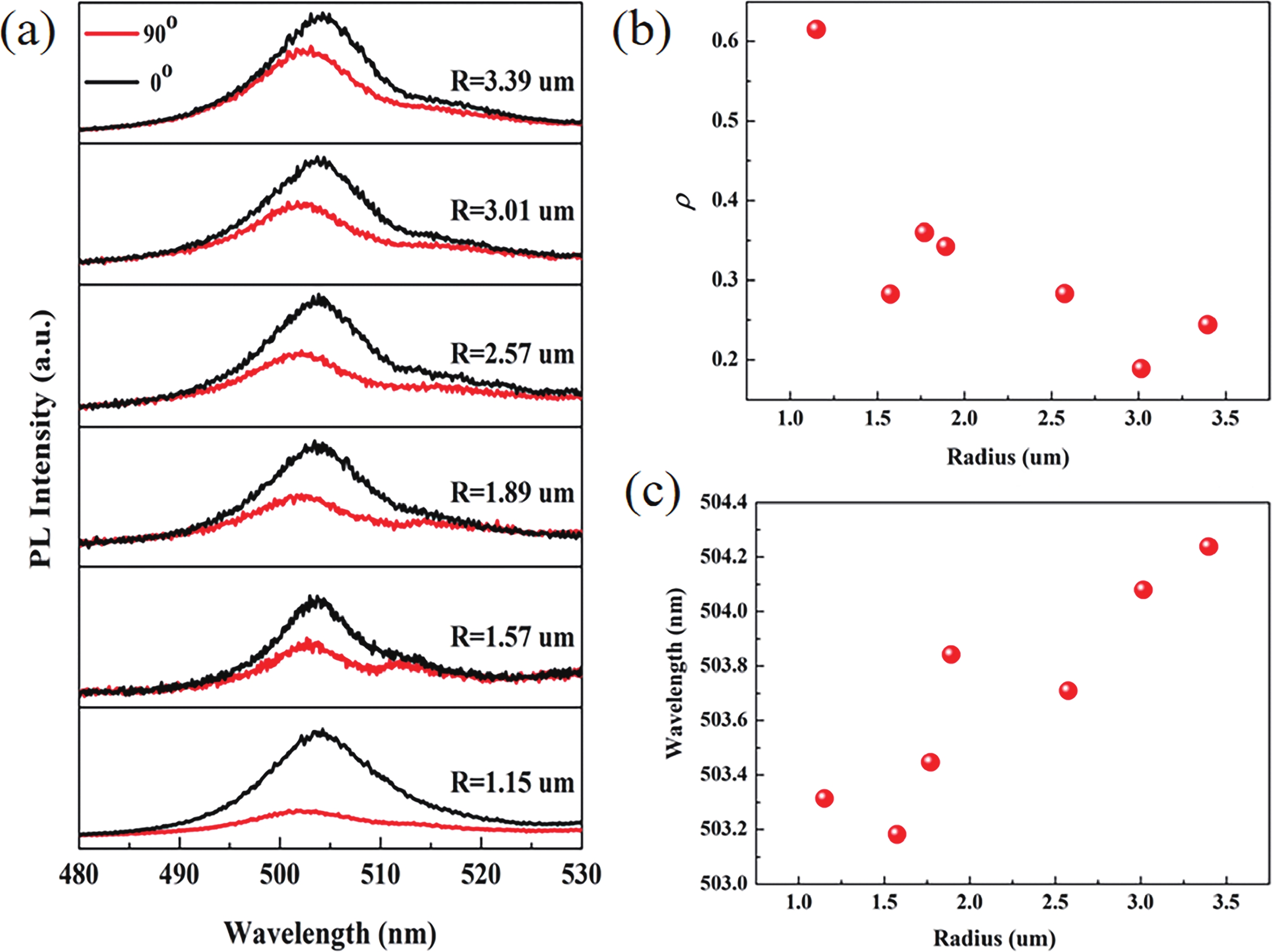
3.3 Nano-comb structure
In 2013, Liu et al.[40] first reported the growth of CdS comb-like branched structures using Sn-catalyzed CVD and confirmed their optical waveguide properties. As shown in Fig. 16, when a continuous-wave GaN laser diode (405 nm) was used to excite the CdS comb-like structure at different positions, the light effectively propagated along the branches and emitted from their tips when the trunk and branch intersection was illuminated. When the central part of the branch was excited, the light emitted more strongly from the branch tip. In both cases, the light entered the trunk, forming a periodic line of light spots, effectively creating a F−P micro cavity on the trunk. Additionally, when the trunk was excited, the light propagated along it and coupled efficiently onto the branches, subsequently traveling to the tips of the branches. These findings provide a novel approach for realizing integrated optical devices with directional one-dimensional wire arrays of hundreds of micrometers in length.
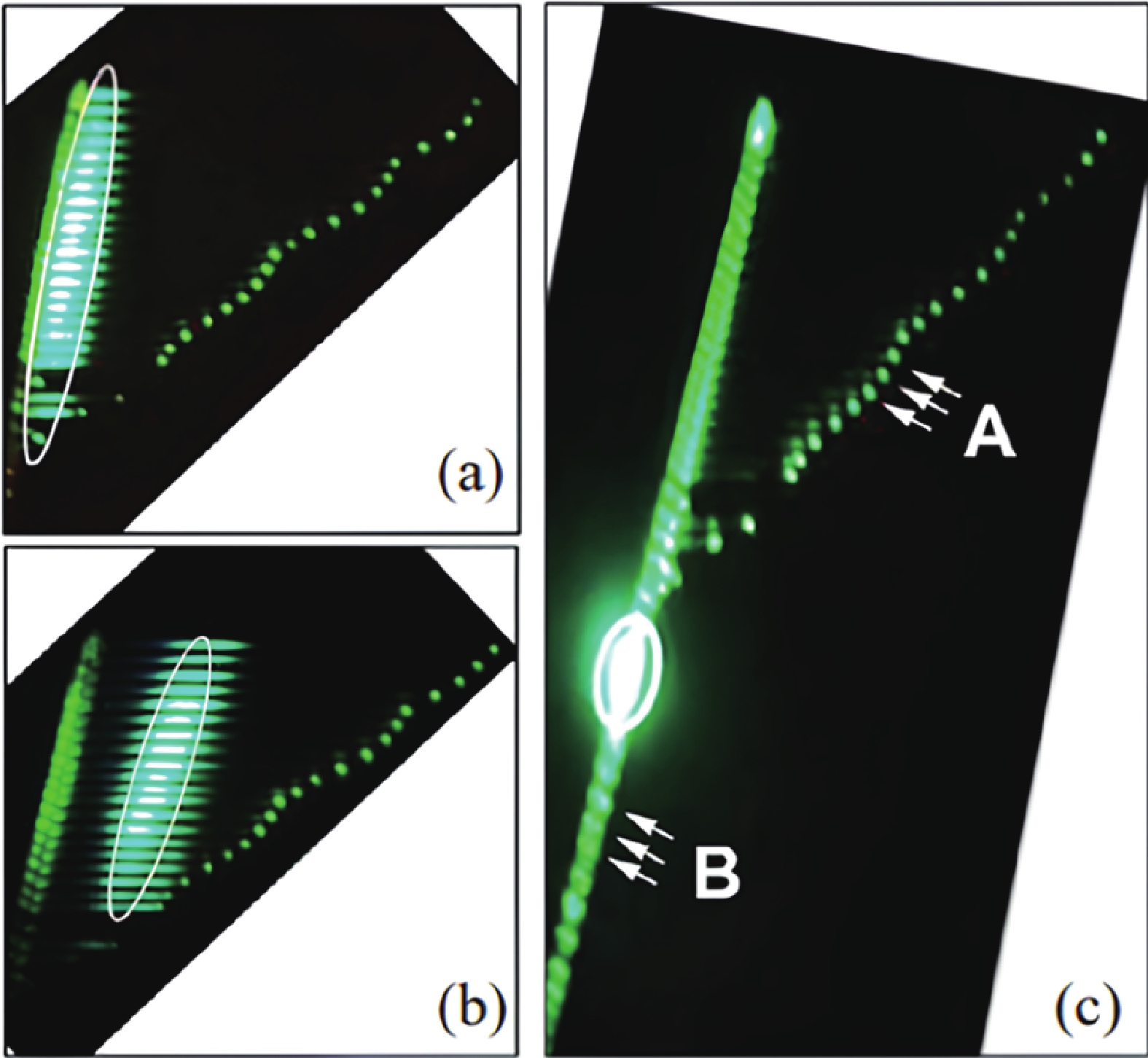
In addition, Guo et al.[59] discovered in 2018 that doping Sn ions in both the trunk and branches of the comb-like structure could significantly enhance its optical waveguide efficiency. Compared to comb-like nanostructures without Sn nanoparticles, samples with Sn nanoparticles encapsulated in the junctions exhibited a much higher number of detected photons and an approximate 20-fold improvement in light transmission efficiency. Subsequently, Song et al.[33] elucidated some of the underlying physical mechanisms using testing methods such as Raman spectroscopy and low-temperature luminescence. Fig. 17(a) presents the Raman spectra of different parts of the comb-like sample, exhibiting three first-order Raman mode peaks at 238, 255, and 303 cm−1, corresponding to A1(TO), E2(high), and LO phonons, respectively. The remaining peaks are attributed to multi-phonon Raman scattering. Under identical measurement conditions, the Raman intensity is stronger in the intersection region compared to other parts. Meanwhile, the Raman spectra of most of the trunk exhibit a slight red shift compared to the branches and intersection region, which is due to the lower Sn doping concentration in the trunk and the highest doping concentration in the intersection region. The incorporation of Sn ions causes changes in the CdS lattice, with grain boundaries or local deformation defects confining more phonons in local spaces, thereby enhancing electron−phonon coupling strength[60]. Fig. 17(b) displays the Raman spectra of comb-like Sn-doped CdS over a temperature range of 78−300 K. As the temperature decreases, the 1TO (210 cm−1) and A1(TO) (234 cm−1) Raman modes exhibit almost no change. However, the E2(high), LO, and 2LO modes shift towards the long-wavenumber side by 4 and 6 cm−1, respectively, while the full-width at half-maximum increases linearly with temperature, indicating a shorter phonon lifetime.
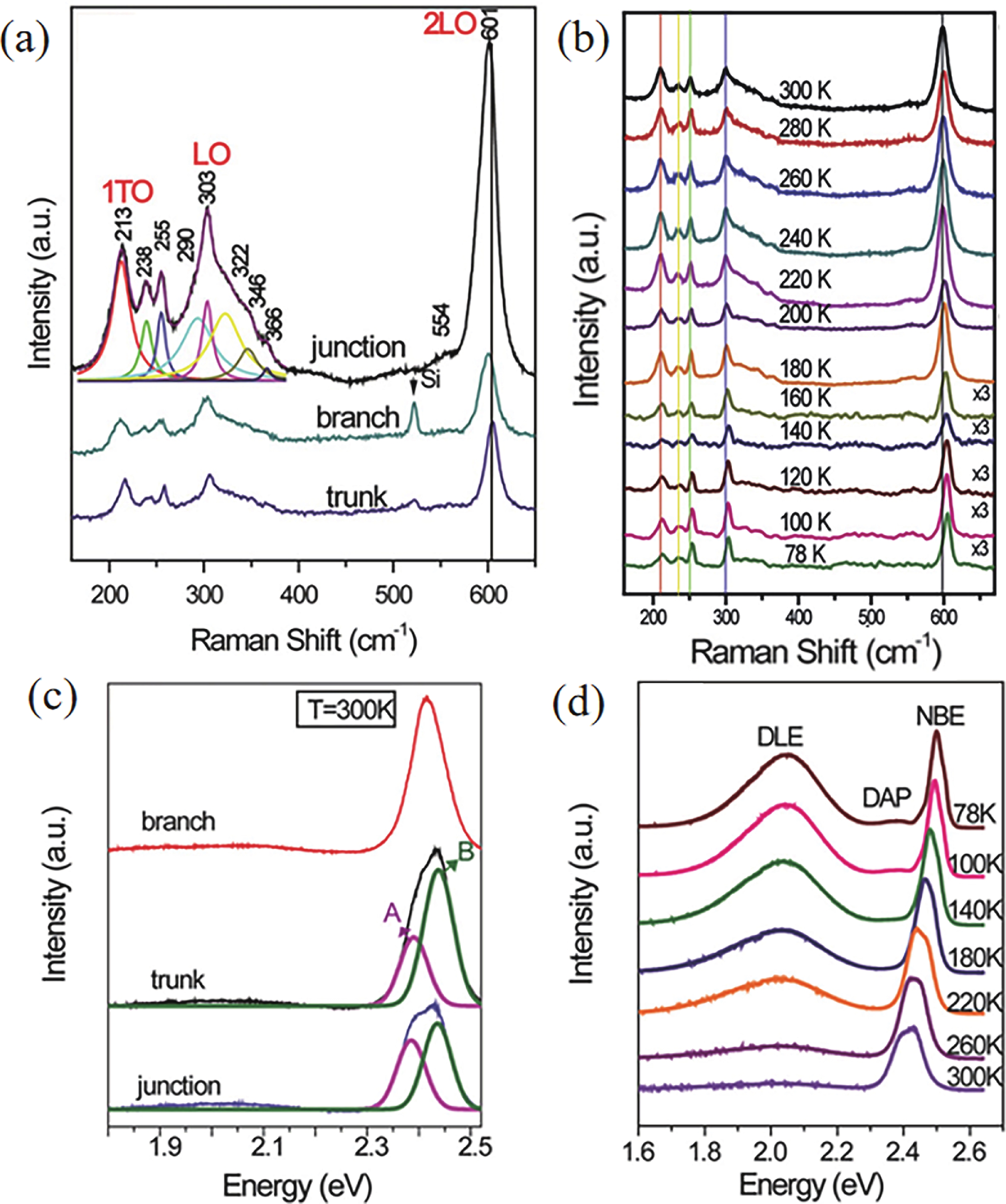
The normalized PL spectra of three different parts of the sample at room temperature are shown in Fig. 17(c), including the near-band-edge emission (NBE) and deep-level emission (DLE). Unlike the spectrum of the branches, the NBE from the trunk and the junction can be fitted with two peaks centered at 2.39 and 2.43 eV, respectively. Fig. 17(d) presents the temperature-dependent PL spectra of the junction over a temperature range of 78 to 300 K. As the temperature increases, the two fitted peaks exhibit red shifts of 110 and 90 meV, respectively, while the DLE remains unchanged. The temperature-dependent bandgap shrinkage is attributed to the expansion of lattice constants as the temperature rises. Additionally, a peak due to donor−acceptor pair (DAP) recombination can be observed at 2.39 eV at 78 K. The intensity of the DAP peak decreases with increasing temperature and disappears at 140 K. The photoluminescence intensity of both NBE and DLE increases rapidly with decreasing temperature due to the reduction of phonon-assisted non-radiative recombination at low temperatures.
Guo et al.[61] demonstrated that these Sn-doped CdS comb-like nanostructures can achieve tunable dual-emission by varying the excitation power intensity. As shown in Figs. 18(a)−18(c), the emission color of the comb-like nanostructure gradually changes from red to green as the laser power increases (2, 6, and 35 mW). The green light originates from near the bandgap edge, while the red light is attributed to defect states. During this transition, the color change occurs first in the branch sections due to their smaller diameters. Even after all sections have completely changed color, the tips of the branches maintain the highest brightness. This finding also provides a method to monitor local laser power and structural diameters. Fig. 18(d) illustrates the specific relationship between spectral intensity and pump power. Below 10 mW, red light emission dominates. As the pump power increases, the green light emission rate increases faster than the red emission rate due to the abundant state density at the green band-edge emission. Saturation is reached when the laser power reaches 35 mW. This saturation is consistent with the mechanism of electron−hole plasma[62]. The tunable dual-color emission property is a characteristic induced by Sn ion doping, as shown in Fig. 18(e), which presents the PL color image of pure CdS comb-like nanostructures. Their emission color does not change with excitation intensity.
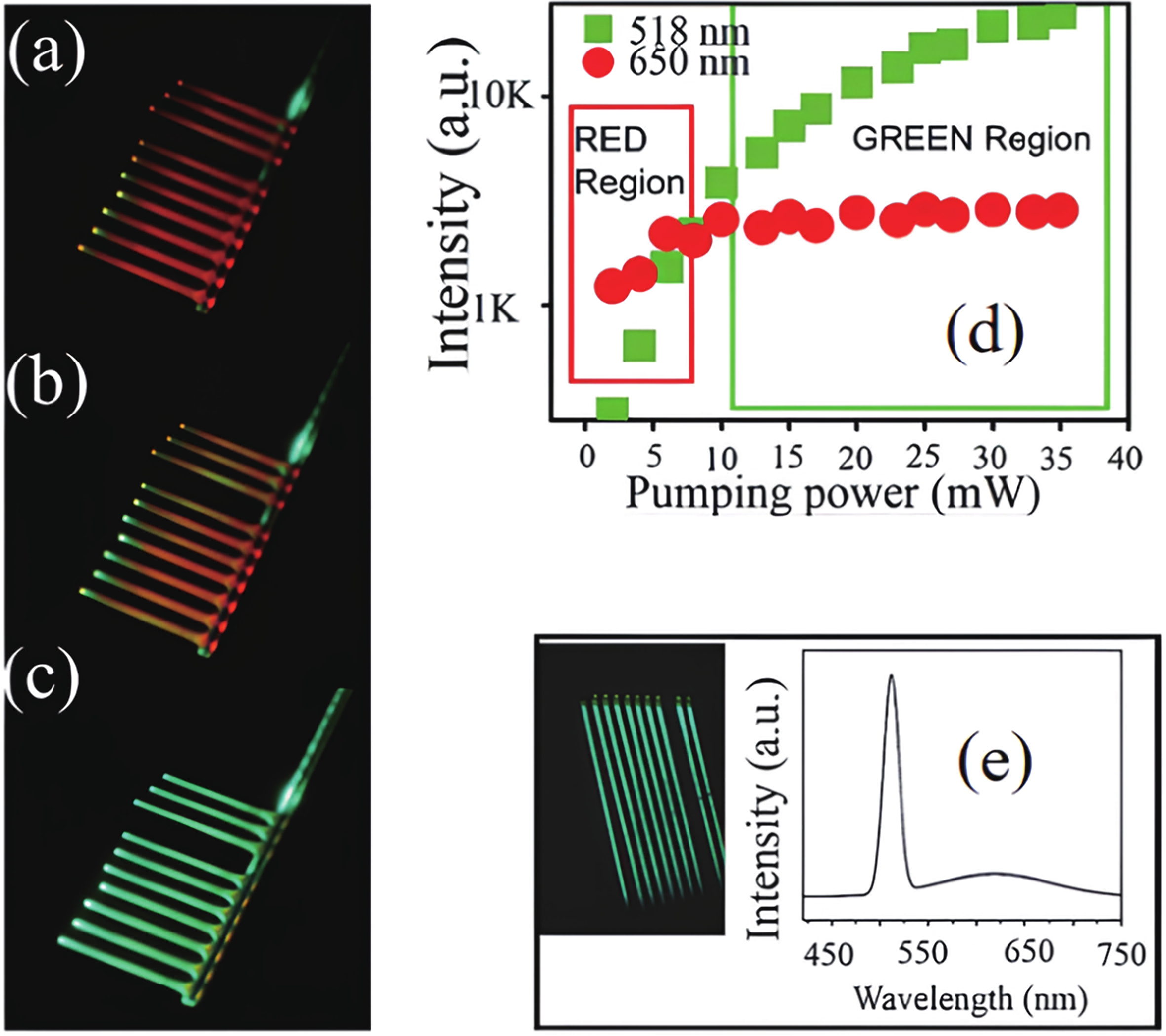
3.4 Other nanostructures
In addition to the aforementioned nano-structures such as lines, cones, and combs, some novel structures have also been discovered in the Sn-doped CdS system, exhibiting rich photophysical properties. In 2016, Zhang et al.[34] prepared a wavy nanobelt Sn-doped CdS structure, specifically characterized by triangular prismatic ridge protrusions alternately formed on both sides of a flat nanobelt surface. These ridge structures can act as high-quality F−P optical cavities, confining waveguide light in the width direction, resonating, and emitting. Their PL spectra also demonstrate that with the increase of pump power, these confined photons can undergo a transition from broad spontaneous emission to sharp stimulated emission.
In 2019, Zhang et al.[32] successfully prepared a nanoporous structure of Sn-doped CdS. They could also control the wall thickness of the porous structure by adjusting the content of Sn in the precursor powder during the preparation process. The luminescence exhibited a series of enhanced microcavity modes within the range of 550−800 nm. Additionally, simulations were conducted to analyze the optical modes and different photonic propagation mechanisms of the Sn-doped CdS nanoporous structures with varying wall thicknesses. Figs. 19(a)−19(c) illustrates the modeled shapes of the CdS porous structures. Fig. 19(d) displays the change in the Q-factor of the optical modes with varying pore radius rhole within the wavelength range of 540−810 nm. For Sn-doped CdS porous structures with small rhole, the internal optical modes maintain the traditional waveguide (WG) mode, as shown in Figs. 19(e) and 19(f). The mode confinement mechanism exhibits high Q-factors in Fig. 19(a), reaching up to 1000. As rhole increases to 0.9 μm, the microcavity modes become confined within the F−P cavity formed between the inner and outer walls, as depicted in Fig. 19(b). Figs. 19(g) and 19(h) present the corresponding typical electric field distributions, where the light waves propagate along the tangential direction of the outer wall edges instead of being reflected. During this transition, the peak positions of the modes exhibit a blue shift, and the Q-factor first decreases and then increases. As rhole continues to increase, the mode confinement mechanism changes to the one shown in Fig. 19(c). The corresponding electric field distributions are presented in Figs. 19(i)−19(l). These modes also exhibit high Q-factors comparable to those of the traditional WG mode but slightly lower than those in Figs. 19(g) and 19(h). Therefore, this novel Sn-doped CdS structure demonstrates that introducing a pore into semiconductor nanowires can yield higher Q-factors than the traditional WG modes in nanowires of the same material. Additionally, adjusting the wall thickness of the nanotubes allows for tuning the Q-factor and optical propagation behavior of the microcavity modes.
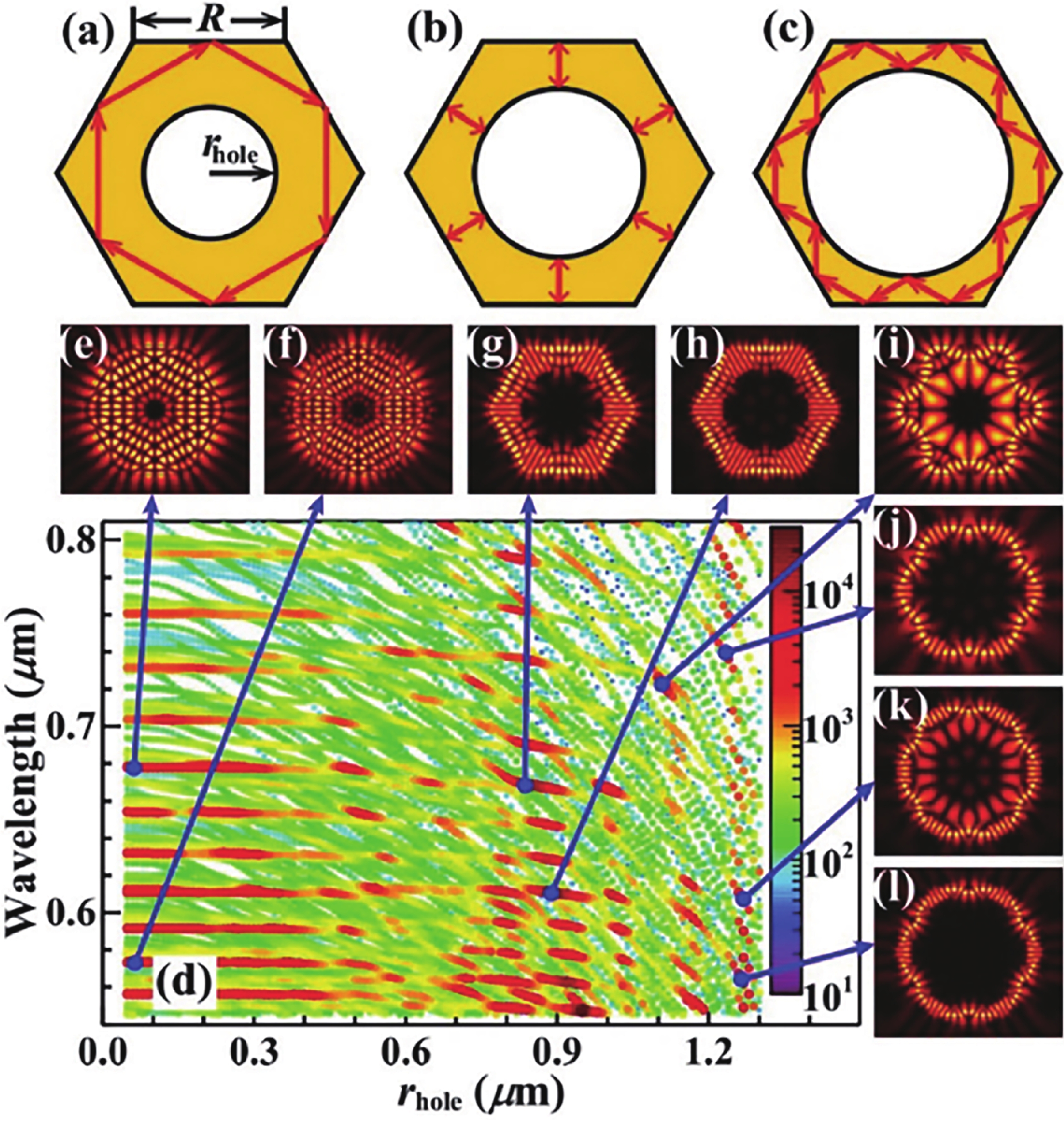
4. Application of Sn-doped CdS nanostructures
4.1 Photodetector
Photodetectors, as components that can convert optical signals into electrical signals, are indispensable in many fields. The quest for photodetectors with higher photocurrent gain, faster response speed, and greater stability remains an ongoing research topic[63]. CdS, a member of the Ⅱ−Ⅵ semiconductor family, exhibits excellent photosensitivity, a direct bandgap of 2.4 eV, high electron mobility of 300 cm2∙V−1∙s−1, and n-type conductive behavior. These properties enable CdS to reduce operating voltage and energy consumption, enhancing both response speed and photosensitivity, thereby finding widespread application in photoelectric sensors[64]. However, the performance of photodetectors based on pure CdS nanostructures is often unsatisfactory. Therefore, how to leverage CdS nanostructures to create high-performance photodetectors remains a worthwhile research question.
In 2014, Zhou et al.[65] investigated the advantages and feasibility of applying Sn-doped CdS nanowires to photodetectors. High-purity CdS exhibits sensitivity to 405 nm light. Notably, Sn-doped CdS nanowires extend this sensitivity to include 532 and 650 nm light, while demonstrating rapid response time, excellent stability, and reproducibility. The doping of Sn not only broadens the spectral response but also enhances conductivity by two orders of magnitude. This enhancement is attributed to the defect states and whispering gallery mode (WGM) microcavities induced by Sn dopants. The photodoping effect, caused by the elevation of the quasi-Fermi level of carriers due to photo-generated excess carriers, leads to an increase in conductivity[66]. Furthermore, the optical WGM microcavity effect effectively enhances the photoresponse performance of Sn-doped CdS nanowires.
In the same year, Zhou et al.[67] successfully designed and fabricated an ultra-high sensitivity and gain white light detector based on a GaTe/Sn : CdS nanosheet/nanowire heterojunction. This heterostructure photodetector demonstrated sensitivity to white light, achieving a responsivity and optical gain of 607 A/W and (1.06−2.16) × 105%, respectively. Additionally, this heterojunction photodetector exhibited good stability and a fast response speed of less than 260 ms. These properties of the GaTe/Sn : CdS mixed PN heterojunction underscore its potential in the field of photosensitive photonic nanodevices.
In 2016, Gou et al.[55] designed and fabricated a UV photodetector based on CdS/CdS : SnS2 superlattice nanowires, as schematically illustrated in Fig. 20. The product was dispersed on a p-type Si substrate with a 200 nm thick SiO2 layer, and then a pair of Ag electrodes with a spacing of 7 μm and a thickness of 30 nm were deposited onto the CdS : SnS2 superlattice nanowires through thermal evaporation. Finally, through comparative experiments, it was found that the photodetector based on Sn-doped CdS superlattice nanowires exhibited a higher photoresponse rate (2.5 × 103 A/W), a higher current on/off ratio (105), excellent external quantum efficiency (8.6 × 105), and a faster response time (10 ms) compared to the detector based on pure CdS. This novel UV photodetector is expected to have promising applications in nano-optoelectronic devices.
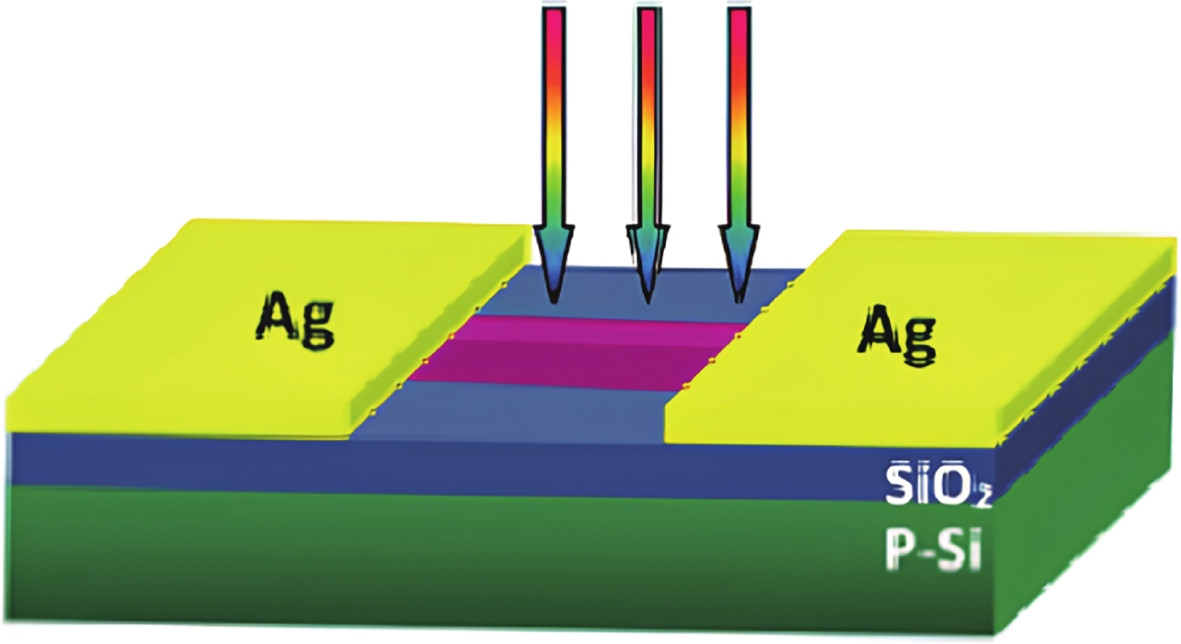
In 2017, Dai et al.[68] combined the advantages of polymer film photodetectors, including low cost, light weight, large detection area, and adjustable spectral response range, with the excellent photoelectric performance of CdS/CdS : SnS2 superlattice nanowires to design a high-performance and flexible photodetector based on a P3HT/CdS/CdS : SnS2 superlattice nanowire hybrid film. The schematic diagram of the energy band structure and carrier transfer for this design is shown in Fig. 21(a), where the two materials form a special p−n junction. When the energy of the incident light is higher than the bandgap of these materials, exciton dissociation occurs in the hybrid device, and a large number of electron−hole pairs are rapidly generated at the interface between the organic P3HT and the inorganic CdS nanowires. Among them, photogenerated electrons can be received by materials with higher electron affinity, while holes can be captured by materials with relatively lower ionization potentials[69]. Therefore, holes and electrons exhibit the migration directions indicated in the Fig. 21. Moreover, this novel p−n heterojunction with a large interface can also increase charge separation and generate higher photoconductive gains through the defect states of CdS nanowires. Figs. 21(b) and 21(c) show the schematic diagram of the flexible hybrid photodetector and a physical image of the hybrid photodetector on a paper substrate, respectively. The designed photodetector ultimately achieves a photoresponse rate of 244 A/W, an on/off current ratio of 1.25 × 103, a fast response time of 12 ms, and a detectivity of 8.7 × 1012 Jones. Furthermore, after being bent 200 times, the photocurrent and responsivity only decrease by 9%. This hybrid photodetector, which exhibits high mechanical flexibility and electrical stability, has broad application prospects in future portable flexible electronic devices. In addition, the same research group proposed a high-quality ternary CdSxSe1−x nanowire system for high-performance photodetectors in the same year, broadening the family of piezoelectric materials and providing a design approach for photodetectors with adjustable performance parameters[70].
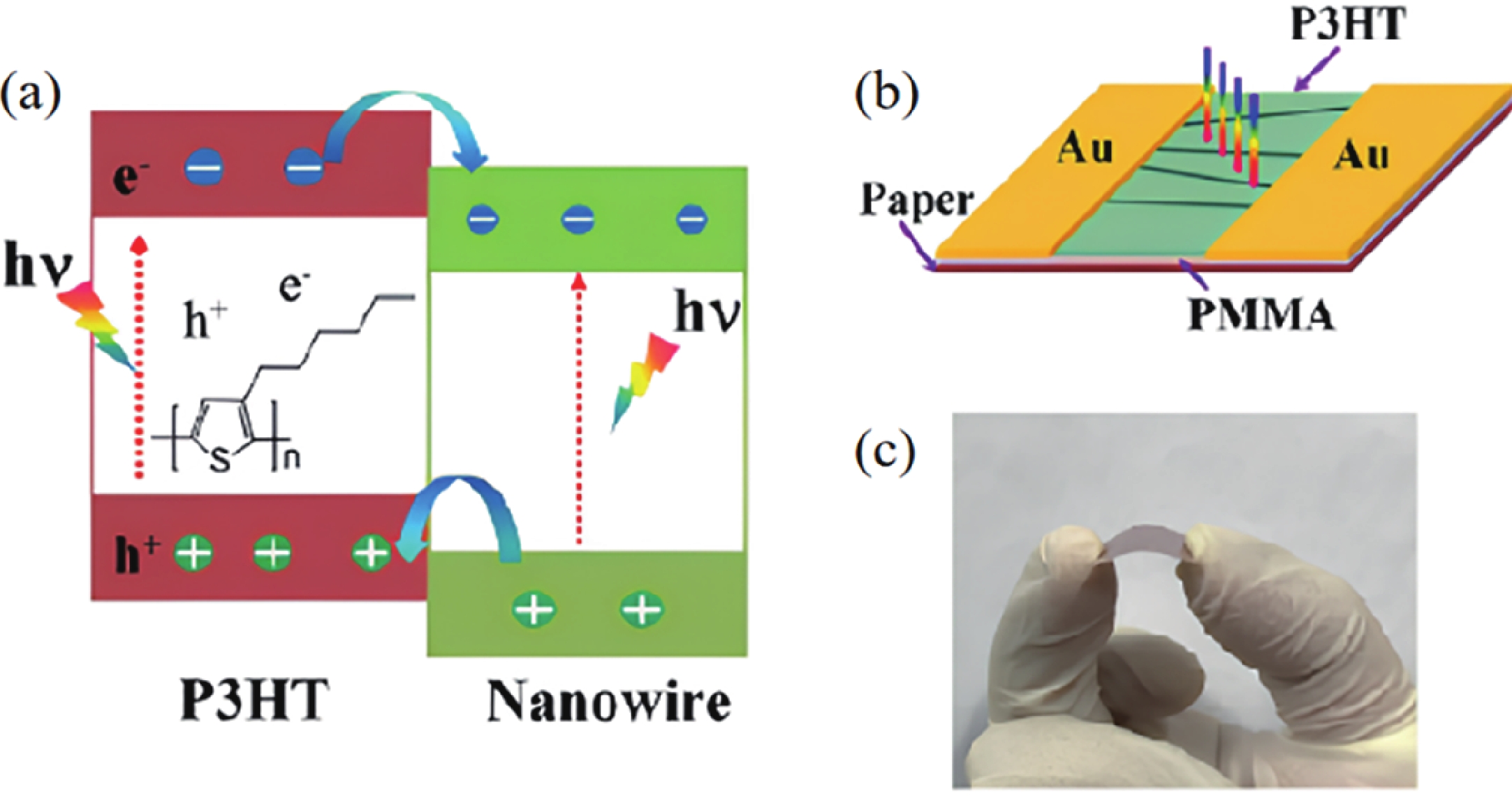
In 2019, Hao et al.[71] designed a high-resolution two-dimensional position-sensitive photodetector based on a Sn-doped CdS comb-shaped nanostructure. As shown in Fig. 22(a), the designed four-quadrant photodetector can be divided into four regions, ⅰ, ⅱ, ⅲ, and ⅳ, and the inset illustrates the circuit to be designed and manufactured. The main principle involves obtaining the resistance in the X and Y directions through the values of current and voltage, and then inferring the position of incident light based on the relationship between distance and resistance. When a light spot irradiates the photosensitive area of the position-sensitive photodetector, the CdS comb-shaped structure can quickly determine the position of the incident laser within hundreds of milliseconds. Fig. 22(b) shows a schematic diagram of the working principle. This design exhibits high sensitivity of 85 and 58 K∙Ω∙μm−1 in the trunk and branch sections, respectively, within a wide frequency band of 310−560 nm, with an optical response of less than tens of milliseconds. Moreover, the unit temperature conductivity change rate of this design is 1.625 × 10−9 A∙V−1∙K−1, which is lower than that of commercial Si-based position-sensitive photodetectors (1.67 × 10−7 A∙V−1∙K−1). Additionally, the design maintains stable performance under low-temperature testing. These results demonstrate that this Sn-doped CdS comb-shaped nanostructure can be applied as a multi-channel device in position-sensitive photoelectric devices with high spatial nanometer resolution.
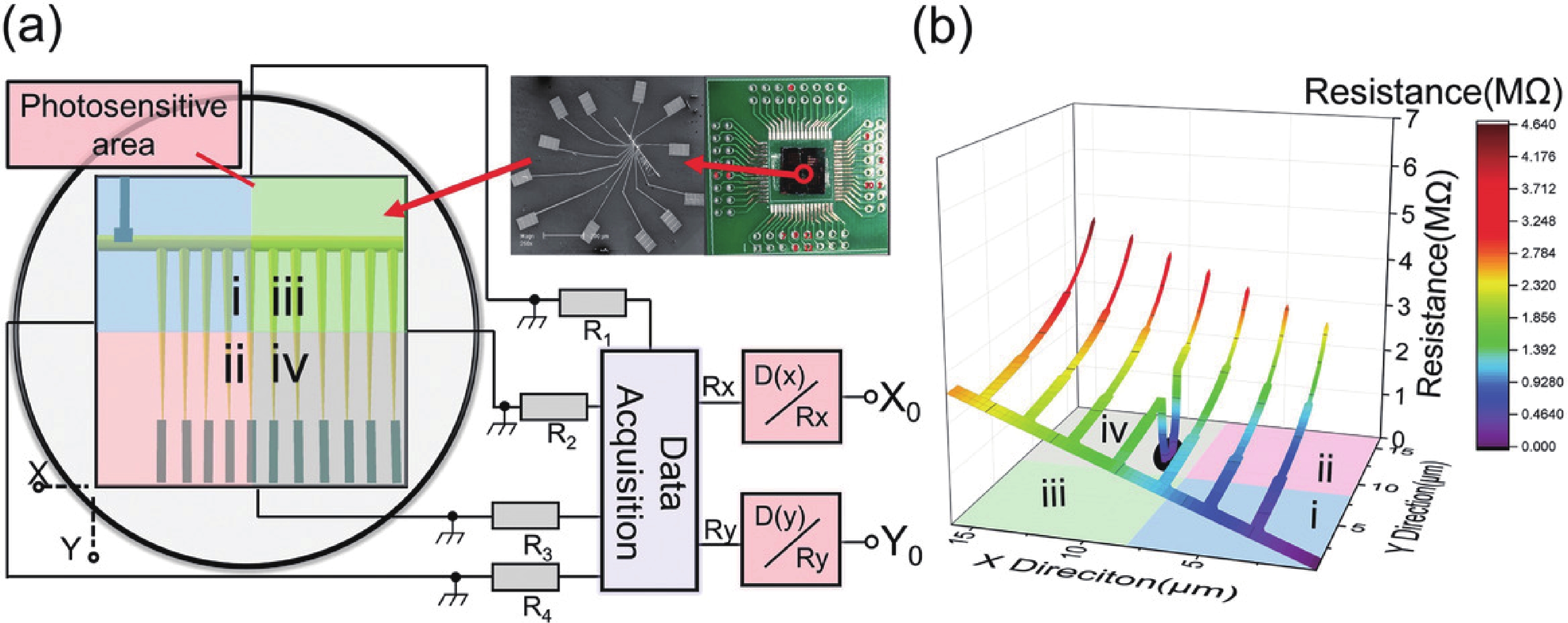
In 2020, Liu et al.[49] fabricated a photodetector based on a single Sn-doped CdS nanowire using photolithography with In electrodes on a Si/SiO2 substrate, as schematically illustrated in Fig. 23. This design also exhibits excellent photoelectric properties, achieving a high responsivity of 51.2 A/W, a high gain of 313.3, stable photoswitching characteristics (trise = 270 ms,tdecay = 310 ms), and multiband response characteristics under low illumination intensity (142 μW/cm2, excited by 405 nm laser).
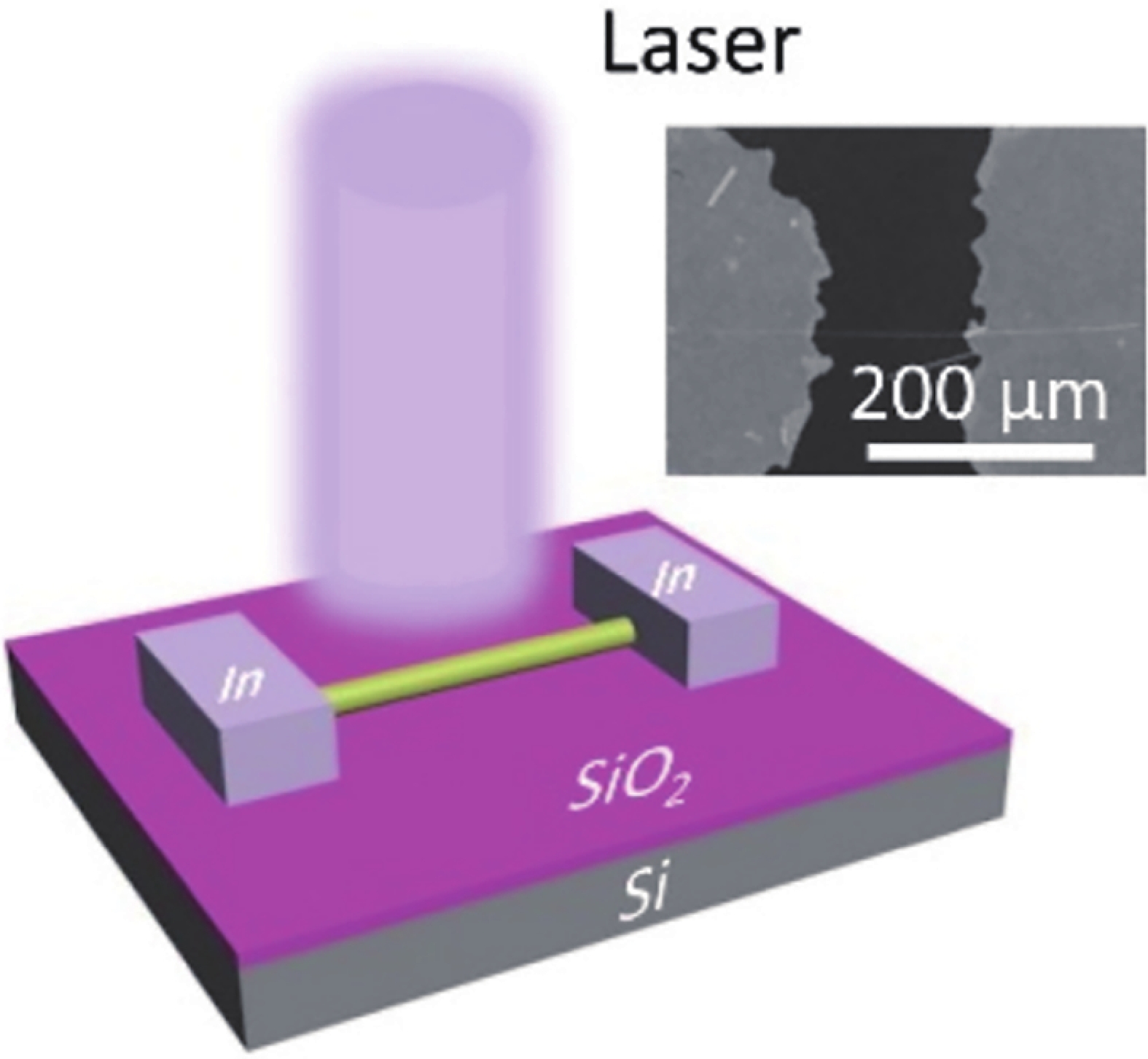
4.2 Laser
Nanoscale laser devices have become a focal area of research due to their prominent advantages in fields such as optical communication, sensing, and integrated optical systems. Low-dimensional semiconductor nanowires or nanoribbons possess resonant cavities that can enhance light and can also act as gain media themselves, thus making them an important choice for designing nanoscale lasers[47, 72]. In 2011, Dai et al.[38] fabricated Sn-doped CdS1−xSex superlattice nanowires that achieved tunable emission wavelengths ranging from 513 to 596 nm, corresponding to colors from green, yellow, orange, to red, by controlling the variable x from 0 to 0.4. Moreover, the periodic optical microcavities within the superlattice structure result in coupled optical cavities, leading to tunable emission in multiple modes. This discovery provides a new material choice for the production of tunable low-threshold lasers, multi-color light-emitting diodes, and other laser devices.
In 2016, Zhang et al.[73] designed a low-threshold single-mode laser using CdS nanoribbons. Figs. 24(a) and 24(c) show the schematic and physical images of the design, respectively. The CdS nanoribbons are sandwiched between dielectric films to form a distributed Bragg reflector (DBR), which helps to lower the threshold of the laser. The principle behind this design is that when the reflection band of the dielectric film DBR matches the band-edge emission of the CdS nanoribbons, the emitted light repeatedly reflects between the two reflectors, as shown in Fig. 24(b), achieving resonance and amplification. This allows the laser to emit light at very low pump power. Additionally, the limited length of the F−P resonant cavity in the thickness direction results in a modal spacing larger than the bandwidth of the optical gain, enabling single-mode operation. Therefore, when the cavity mode of the DBR is adjusted to match the emission band of the CdS nanoribbons, single-mode laser output can be achieved. The mode wavelength can also be tuned by varying the thickness of the CdS nanoribbons. Fig. 24(d) presents the laser spectra of CdS nanoribbons with thicknesses of 400, 230, 304, 110, and 316 nm embedded in the same DBR cavity. The wavelength of the laser mode can be continuously modulated within the range of 498−520 nm, covering the entire band-edge emission band of CdS. This low-threshold single-mode laser has potential applications in on-chip optical communication, signal processing, and integrated photonics.
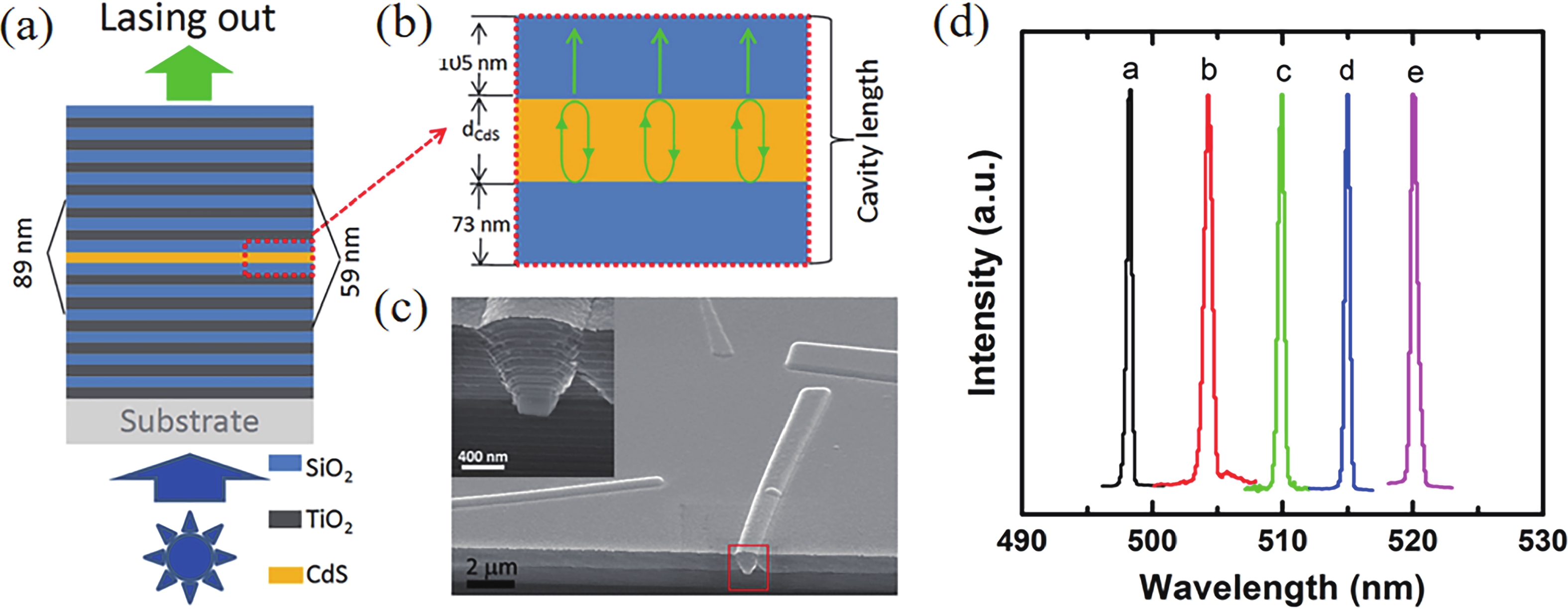
In the same year, Zhang et al.[34] also demonstrated a nano-laser array based on CdS nanoribbons. The periodically protruding structures of the prepared nanoribbons provided resonant cavities that confine light in the width direction. Under the excitation of pumping lasers, these confined lights can achieve lasing, forming a laser array. Fig. 25(a) shows the image of such nanoribbons under pulsed laser irradiation above the lasing threshold, where the emission points of the lasers can be observed. The PL spectra of these detection points are presented in Fig. 25(b), revealing that they are all very narrow laser modes. This novel nanoribbon structure offers a new design for integrated optical microcavities, facilitating the realization of high-density photonic integrated circuits.
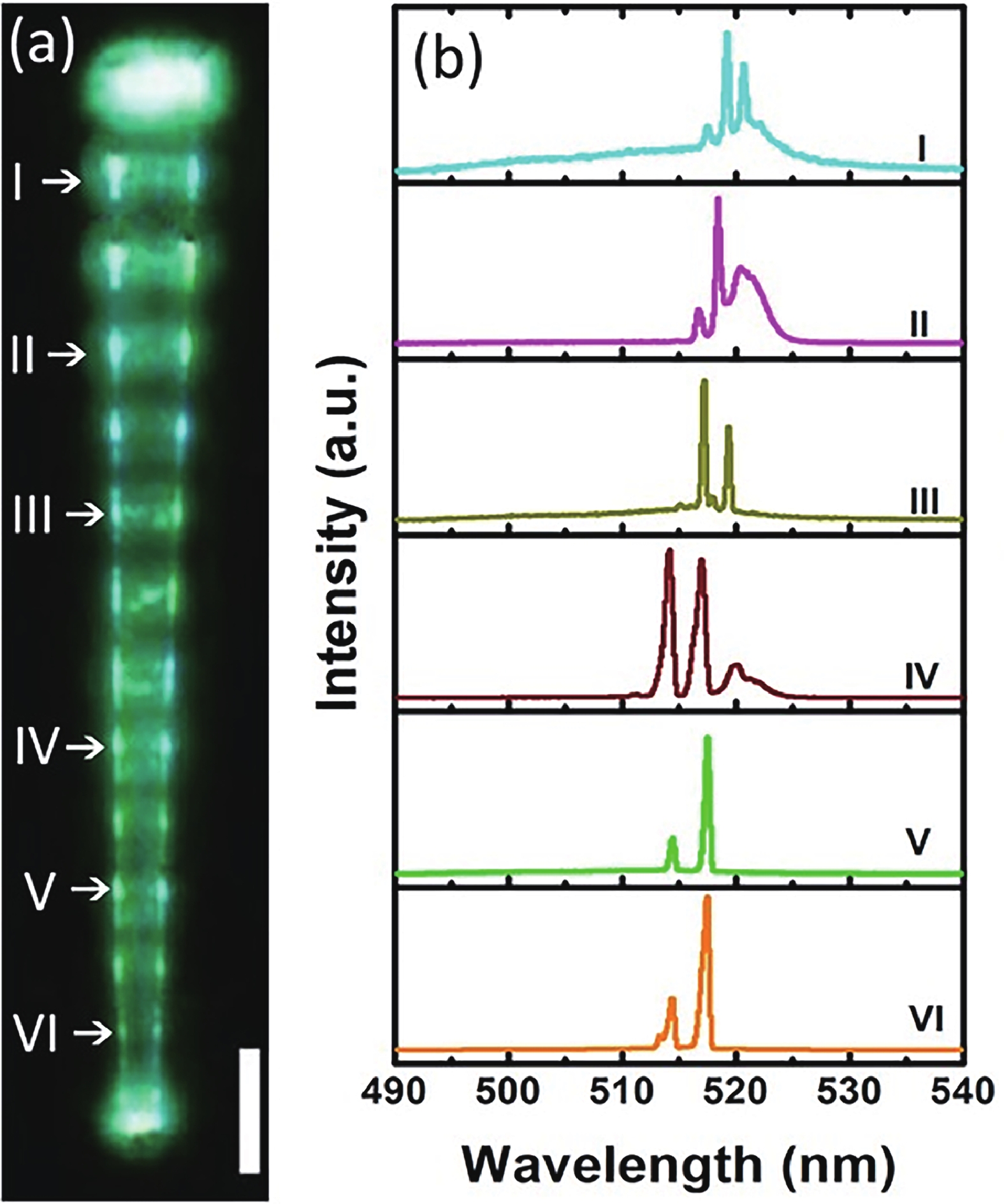
In 2019, Zou et al.[52] found that it was difficult to achieve laser emission in Sn-doped CdS nanowires using continuous-wave lasers. This was due to the significantly lower power of continuous-wave lasers compared to pulsed lasers, which prevented the occurrence of population inversion. Additionally, the non-negligible thermal effects resulting from the continuous interaction between light and nanostructures greatly hindered the generation of laser light. Based on these findings, Zou's team excited a single Sn-doped CdS nanowire using a 405 nm continuous laser at 80 K. The local plasma formed by Sn ions of different valence states and the enhanced cavity effect at low temperatures significantly enhanced the emission light, enabling the achievement of laser emission from both the band-edge and bandgap whispering gallery modes (WGMs). This nanowire laser provides an effective approach for the development of low-threshold, temperature-tunable microscale lasers.
4.3 Other application
In addition to the aforementioned application directions, Sn-doped CdS micro-nano structures can also exhibit innovative effects in other areas of application. In 2022, Dai's research group[74] addressed the challenge of precisely arranging one-dimensional semiconductor nanowires with excellent photoelectric properties into desired structures, such as arrays or grids, when assembled into two-dimensional devices. They designed a method for automatically aligning nanowires to a desired direction. By utilizing complex amplitude beam shaping techniques in two gratings with a gap, they designed auto-alignment beams with controlled intensity and phase distributions. Considering the forces acting on the nanowires, including gravity, viscous force, buoyancy, intensity gradient force, and phase gradient force, the gravity and buoyancy are approximately balanced and can be neglected. Therefore, the position of the nanowires was adjusted by controlling the auto-alignment beam to influence the intensity gradient force and phase gradient force in combination with the inherent viscous resistance. As shown in Fig. 26, this method was used to automatically align three disordered CdS nanowires to be approximately parallel to each other. This process can be repeated to achieve more complex two-dimensional devices. Compared to traditional nanowire manipulation and assembly techniques, this technique avoids photolithography, is more convenient, and provides a lower-cost and simpler approach for the construction of novel nanophotonic devices.
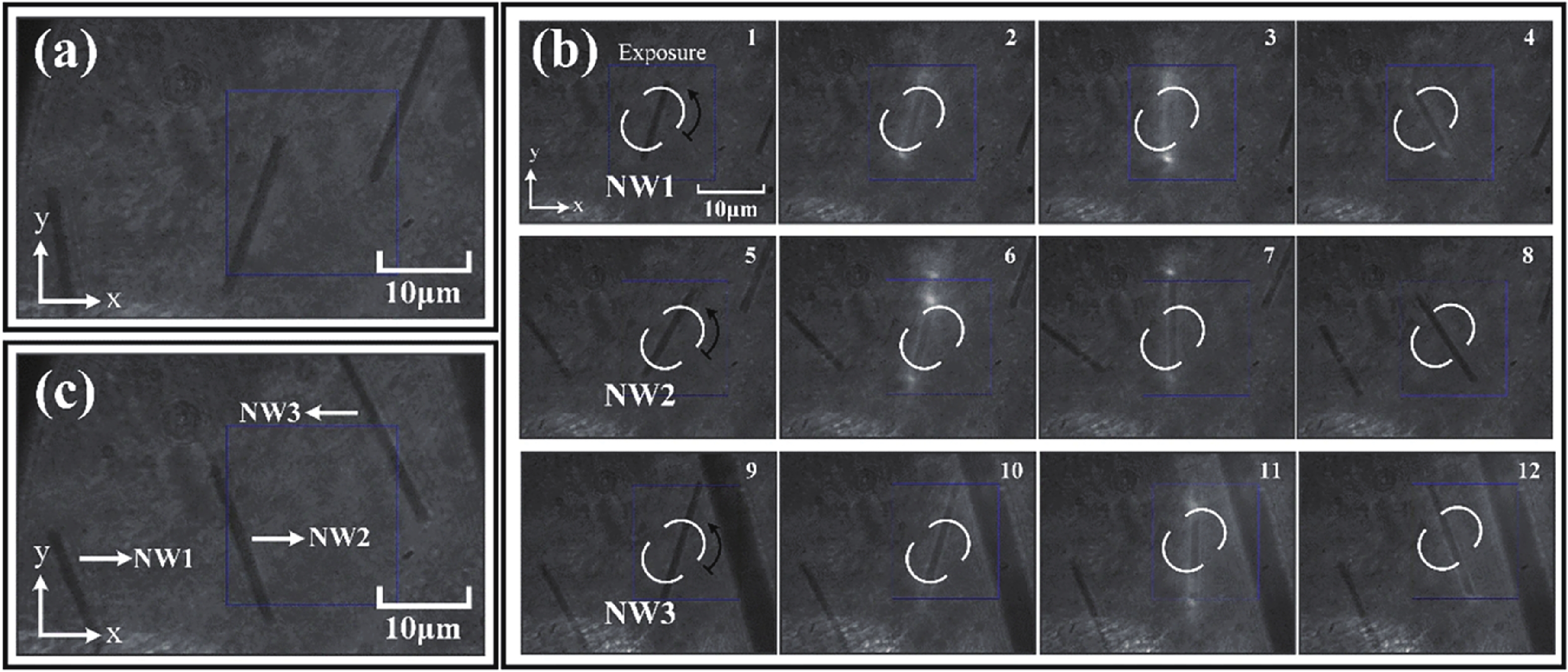
In 2023, Liu's research group[75] proposed the application of Sn-doped CdS nanocomb structures in establishing a photonic barcode-based anti-counterfeiting label in the two-dimensional domain. In this barcode application, the position of each line is fixed, while the width of each line, reflecting the encoding capability, is primarily modulated by temperature and pump laser power through the modulation of PL intensity and the relative luminescence intensity at different branch ends. Figs. 27(a) and 27(e) show the PL spectra of the main trunk at 300 and 78 K, respectively. The emission band at 513 nm and the peak at 604 nm correspond to near-band emission and deep-level emission[76], respectively. The deep-level emission is caused by surface defects introduced by S vacancies during the growth process[77]. At low temperatures, the lattice scattering effect weakens, leading to a sharp increase in the intensity of deep-level emission and a larger luminescent region. Figs. 27(c) and 27(g) depict the luminescence intensity distribution curves at different branch ends after light propagates from the main trunk to the branches. The barcode at 300 and 78 K is achieved through the combination of PL intensity and the luminescence intensity distribution at different branch ends, as shown in Figs. 27(d) and 27(h), respectively.
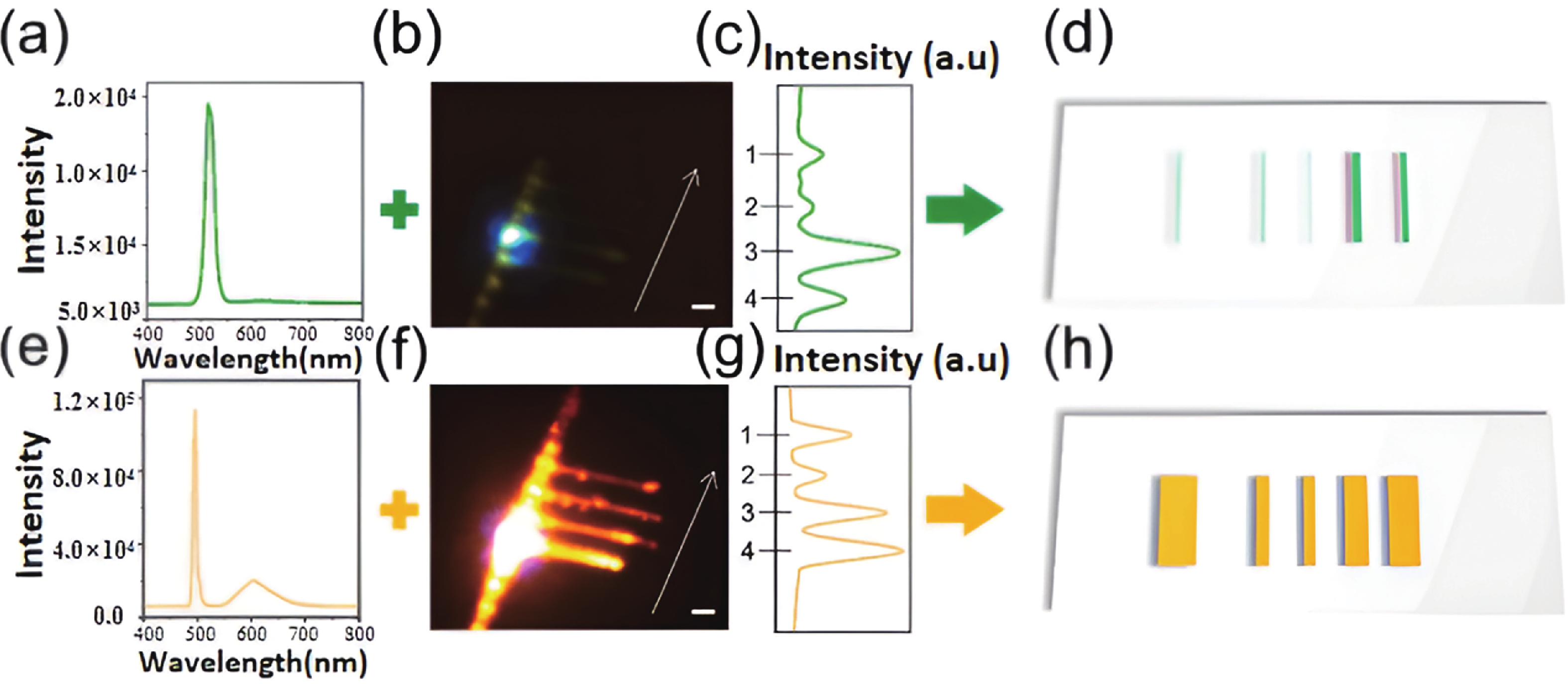
In addition, the encoding capability is further enhanced by incorporating the variable of excitation power regulation. As the laser intensity increases, the luminescent color of the comb-like Sn-doped CdS nanostructure changes from red to green. Figs. 28(a) and 28(b) show the true images of the two colors, while Figs. 28(c) and 28(d) are the corresponding PL spectra. This tunable luminescent color property can also improve the encoding security of the designed photonic barcode. Moreover, the emission intensities of defect states and band edges vary under different excitation powers. Finally, as shown in Fig. 28(e), various photonic barcodes can be achieved by adjusting both temperature and excitation power. Compared to traditional techniques, this photonic barcode exhibits higher identifiability and confidentiality, demonstrating great potential in the development of novel anti-counterfeiting labels.
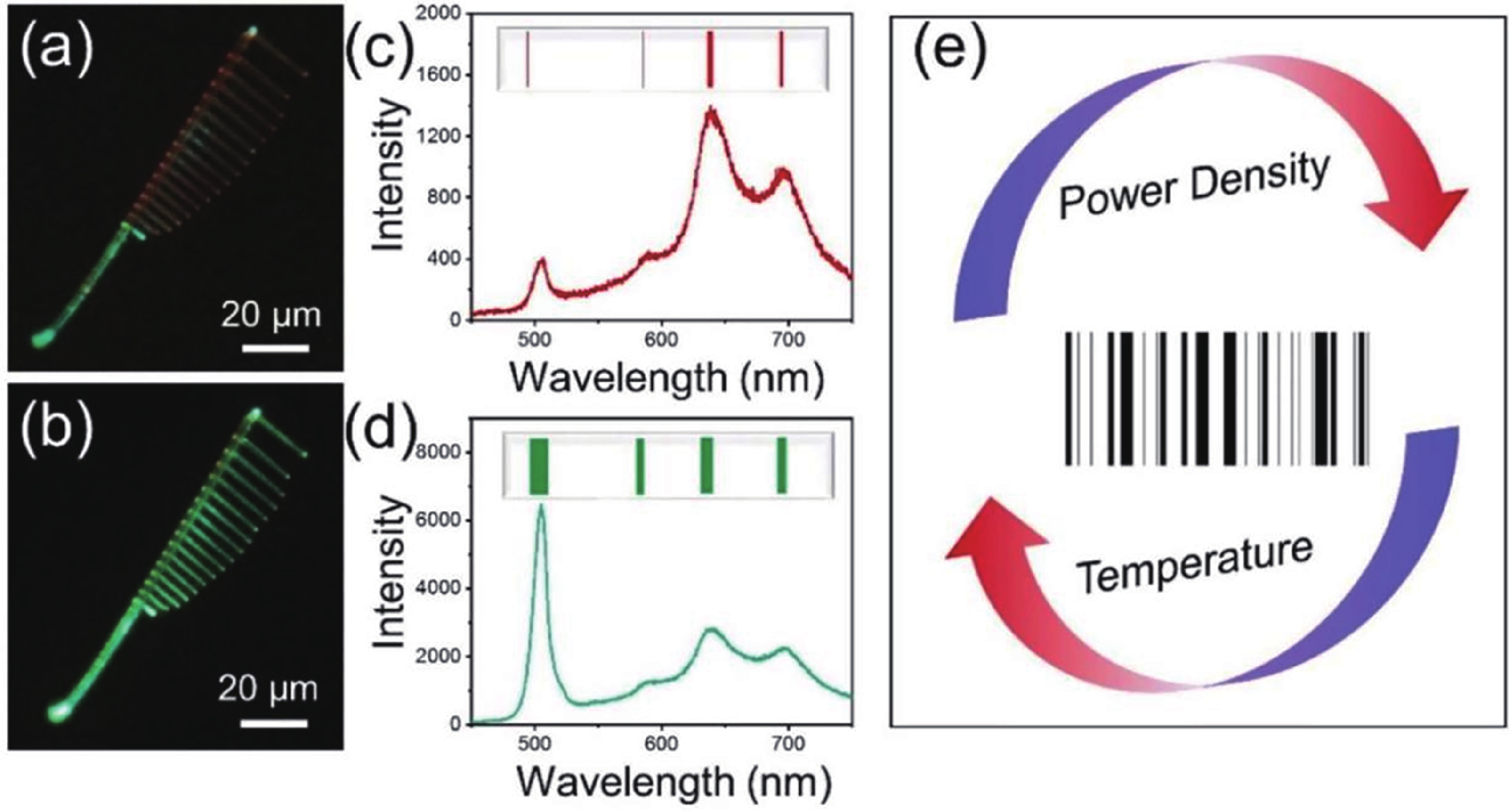
5. Conclusions and outlook
This article summarizes the impact of various growth conditions during the preparation of different micro-nano structures of Sn-doped CdS, including nanowires, microcones, nanobelts, comb-like structures, core-shell structures, and superlattices, in recent years. It also presents the research progress on the basic optical absorption characteristics, luminescent properties, photoconductive abilities, electronic structures, exciton-polariton, and other optical-physical properties of these structures. Furthermore, the article explores their application prospects in the fields of photoelectric sensing, laser devices, and other areas.
The Sn-doped CdS nanostructures are primarily synthesized through the Sn-catalyzed CVD growth method, where all the growth condition parameters play a crucial role in determining the morphology and ion doping level of the nanostructures. Precise control over the synthesis of Sn-doped CdS nanostructures with different characteristics can be achieved by varying various growth conditions, including growth temperature, reaction time, heating rate, mass ratio of CdS to SnO2, and gaseous environment.
In terms of photophysical properties, researchers have conducted meticulous experiments and theoretical analyses to delve into the photophysical properties of various Sn-doped CdS micro-nano structures through various optical characterization techniques such as absorption spectroscopy, fluorescence spectroscopy, Raman spectroscopy, and corresponding polarization spectroscopy. The introduction of Sn effectively modulates the band structure of CdS, thereby enhancing its light absorption range and intensity. micro-nano structures of Sn-doped CdS with different morphologies also exhibit unique optical properties, such as enhanced luminescence efficiency, optimized photoconductive performance, diverse optical microcavities, and unique exciton-polariton phenomena. These discoveries not only enrich our understanding of the photophysical properties of Sn-doped CdS but also provide strong support for subsequent application research.
In terms of applications, Sn-doped CdS micro-nano structures have attracted significant attention due to their excellent photoelectric properties. In the field of photoelectric sensing, the high sensitivity, high current switching ratio, and fast response characteristics of these materials make them ideal sensing elements for detecting various optical signals and chemicals. Additionally, in the field of laser devices, the outstanding luminescent performance and stability of Sn-doped CdS, along with the diverse microcavity modes of different structures, render it a potential material for the manufacture of efficient, stable, and diverse lasers. Furthermore, with the deepening of research, some special Sn-doped CdS micro-nano structures have gradually exhibited broad prospects for applications in other fields such as complex two-dimensional device integration, photocatalysis, anti-counterfeiting, and more.
However, despite the excellent photophysical properties and broad application prospects of Sn-doped CdS micro-nano structures, as well as the significant progress made in these areas, there are still some challenges and issues to be addressed. For instance, how to further optimize the doping process and preparation conditions, precisely adjust the growth condition parameters, and more accurately control the synthesis of different nanostructures in order to enhance the performance and stability of the materials. Additionally, how to fabricate new structures with even better performance, how to further explore the regulation mechanism of Sn doping on the photophysical properties of CdS with different structures to achieve more precise performance control, and how to expand the application of Sn-doped CdS in more fields to meet the needs of different areas are also crucial questions to be answered.
With the rapid development of nanotechnology and the continuous emergence of novel preparation methods, research on the photophysical properties and applications of Sn-doped CdS micro-nano structures will continue to make breakthroughs. We anticipate addressing the current issues and challenges through further research and technological innovation, promoting the widespread application of Sn-doped CdS micro-nano structures in the field of optoelectronics. Additionally, we hope that research in this area can intersect and integrate with other related fields, jointly driving scientific and technological progress and societal development.
Acknowledgements
This work was supported by National Natural Science Foundation of China (52275551) and Shanxi Scholarship Council of China (2021–117).